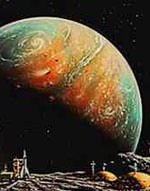
Image credit: NASA
Locally, Earth has its habitable extremes: Antarctica, the Sahara desert, the Dead Sea, Mount Etna. Globally, our blue planet is positioned in the solar system’s habitable zone, or ‘Goldilocks’ region where the temperature and pressure are just right to support liquid water and life. Across the borders from this goldilocks zone orbit our two neighbors: the runaway greenhouse planet, Venus–which in goldilocks’ terms is ‘too hot’–and the frigid red planet, Mars, which is ‘too cold’.
With an average global temperature of -55 C, Mars is a very cold planet. The standard models for warming Mars raise this average temperature with greenhouse gases first, then plant cold-adapted crops and photosynthetic microbes. This terraforming model includes various refinements such as orbital mirrors and chemical factories which pour out fluorocarbons. Eventually with the help of biology, industrialization, and time, the atmosphere would begin to get thicker (the current martian atmosphere is 99% thinner than the Earth’s). To terraform Mars, depending on the choice and concentration of greenhouse gases used, can take many decades to centuries before an astronaut might begin to lift a visor and for the first time, breathe martian air. Such proposals would initiate the first conscious effort at planetary engineeering, and aim to change the global environment into one less hostile to life as we know it terrestrially.
Another version to these global changes is a local one familiar to those who have trekked the Sahara. Occasionally life blossoms into a desert oasis. A local strategy to change Mars, according to biologist Omar Pensado Diaz, director of the Mex-Areohab project, can best be compared to transforming Mars one oasis at a time. The minimum size of the oasis extends to the diameter of a dome-shaped plastic cover, much like a greenhouse with a space heater. In this way, microterraforming is the smaller alternative for a planet that otherwise is an open system leaking to space. Diaz contrasts the way a physicist might change Mars with industrial tools to the hothouse methods of a biologist.
Diaz talked with Astrobiology Magazine about what it might mean to remodel Mars with tiny stadiums, until they grow into lush, desert oases.
Astrobiology Magazine (AM) : Would it be correct to conclude that you are studying the differences between a global and local terraforming strategy?
Omar Pensado Diaz (OPD): I am looking forward to integrating the models, rather focusing on their differences. Global terraforming, or warming a planet with super greenhouse gases, is a strategy or model conceived from the perspective of physics; while the model I propose is seen from a biological point of view.
I am talking about a model called microterraforming, which will be possible with a tool named the Minimal Unit of Terraforming (MUT). The concept of a Minimal Unit of Terraforming is explained as an ecosystem running as the fundamental unit of nature. A MUT comprises a group of living organisms and their physical and chemical environment where they live, but applied to the development of a biological colonization and remodeling process on Mars.
An artist’s conception of how a terraformed Mars, with an ocean spanning most of its northern hemisphere, might look from orbit.Mars, as terraformed by Michael Carroll. In 1991 this image was used on the front cover of the ‘Making Mars Habitable’ issue of Nature.
Technically speaking, it is a pressurized dome-shaped greenhouse that would contain and protect an interior ecosystem. This complex would not be isolated from the surroundings; on the contrary it would be constantly in contact with it, but in a controlled way.
What is important is gas exchange between the MUT Units and the Martian environment, so the ecosystem itself has a dramatic role. The objective of this process is to generate photosynthesis. Here is where we must consider plants as covering the surface and chemical factories processing the atmosphere.
AM: What would be the advantages of working locally, using your model of an oasis in a desert? By biological analogy to a fundamental terraforming unit, do you mean like how biological cells have an internal equilibrium, but also exchanges with an external one that differs for the whole host?
OPD: The advantages I find in this model are that we can initiate a terraforming process faster, but in stages, that is why it is microterraforming.
But the major and most important advantage is that we can make plant life begin to participate in this process with the help of technology. Life is information and it processes the information around it, beginning an adaptation process to the inner conditions of the unit. Here we maintain that life has plasticity and that it not only adapts to the surrounding conditions, but also it adapts the environment to its own circumstances. In the language of genetics, this means that there are an interaction between the genotype and the environment, producing the adaptation of phenotypical expressions to the dominant conditions.
Now, in a small environment such as a Unit with a diameter of approximately 15 or 20 yards, we could have a much warmer environment than outside the Unit.
AM: Describe what a Unit might look like.
OPD: A transparent, plastic-fiber, double-layered dome. The dome would generate a greenhouse effect inside that would raise significantly the temperature during the daytime and would protect the inside from low temperatures at night. Furthermore, the atmosphere’s pressure would be higher inside by 60 to 70 millibars. That would be enough to allow the plants’ photosynthetic processes as well as liquid water.
In thermodynamic terms, we are now talking about a lack of equilibrium. In order to reactivate Mars, we need to create a thermodynamic disequilibrium. The Unit would generate what is needed first, like ground degassing from temperature differences. Such process is an objective along with the path to a global strategy.
Strictly speaking, the Units would be like carbon dioxide capturing traps; they would release oxygen and generate biomass. The oxygen would then be released to the atmosphere periodically. A valve system would release gases to the outside and once the inner atmospheric pressure had decreased up to 40 or 35 milibars, the valves would close automatically. And others would open and, by suction, gas would get inside the Unit and the original atmospheric pressure would level off. This system would not only allow the release of oxygen but also the release of other gases.
AM: In such an oasis model, it is an open system, but would it have no effect on regional conditions. In other words, would local leakage get diluted, and in those cases, how is microterraforming different from just operating greenhouses?
OPD: The greenhouses–in this case the Minimal Unit of Terraforming–are thought to begin a gradual change on Mars. The difference depends on its range of action, since that’s where the microterraforming process begins. Besides, it depend on how you look at it, because with this method we are trying to repeat the evolution pattern that once was successful on Earth, in order to transform the planet’s atmosphere into another and to make Mars enter in a stage of thermodynamic disequilibrium.
The major advantage is that we can control a terraforming process at a micro-scale; we can turn Mars into a similar place to the Earth faster and make it interact with the surrounding environment at the same time. That is the most important aspect of it: to get ahead with faster processes. As I said before, the idea is to follow the same evolution pattern that developed on Earth soon after photosynthesis appeared. There were terrestrial plants that remodeled and terraformed the Earth, generating carbon dixoide from the surface and distributing it to the atmosphere that existed at that time.
Drs. Chris McKay and Robert Zubrin presented an interesting model that proposes to collocate three large orbital mirrors. The mirrors would reflect the Sun’s light to the south pole of Mars and sublimate the dry ice (carbon dioxide snow) layer in order to increase the greenhouse effect and then accelerate the planet’s global warming.
Such mirrors would be the size of Texas.
I think that if the same infrastructure used in those mirrors were instead used to build domes for a Minimal Unit of Terraforming over the Martian surface, we would be generating higher degassing rates and oxygenating the atmosphere faster. In addition, part of the surface would be warmed anyway, since the Units would hold solar heat, not reflect it from the surface.
The lack of liquid water for the ecosystems inside the Units is debatable; however, a variant of a proposal by Dr. Adam Bruckner from the University of Washington, can be used. It consists on using a zeolite (mineral catalyst) condenser; then, extracting water from the moisture of incoming air. Water would pour inside daily. Again, we would be activating some stages of a hydrological cycle, capturing carbon dioxide, releasing gases to the atmosphere and making the surface a more fertile ground. We would be doing an accelerated terraforming on a very small part of Mars, but if we put hundreds of those Units, the degassing effects over the surface and atmosphere will have planetary repercussions.
AM: When closed biospheres have operated on Earth like Biosphere 2, problems arose with–for instance–oxygen loss due to combination with rock to form carbonates. Are there examples today of large-scale, self-sustaining systems on Earth?
OPD: Large-scale, self-sustaining systems built by humans? I don’t know any, but life itself is a self-sustaining system that takes from the surrounding environment what it needs to work.
That was the problem of closed biospheres, they were not able to make a feedback circuit as it happens on Earth. Furthermore, the system I propose would not be closed; it would interact with the environment of Mars in intervals, by releasing part of what would have been processed by the action of photosynthesis while incorporating new gases. The Minimal Unit of Terraforming will not be a closed system.
If we take into account James Lovelock’s ‘Gaia theory’, we could consider Earth as a large-scale, self-sustaining system, because the biogeochemical cycles are active–a situation that is not happening today on Mars. A large portion of its oxygen is combined with its surface, giving the planet an oxidized character. In this sense, inside the Minimal Unit of Terraforming, the biogeochemical cycles would be reactivated. These domes would liberate oxygen and carbonates, among others, so the release would begin to flow gradually to the planet’s atmosphere.
AM: The quickest method often cited for global terraforming is to introduce fluorocarbons into the Martian atmosphere. With small percentage changes, big temperature and pressure changes follow. This relies on solar interaction. Would a closed bubble have this mechanism available, for instance if ultraviolet light is not penetrating into the domes?
OPD: We are talking about an alternate way from that–not using fluorocarbons and other greenhouse gases. The method we propose captures carbon dioxide for biomass increase, liberates oxygen and inner heat storage, all to generate a carbon dioxide degassing inside the Unit. Other gases trapped in the ground today would be released to the Martian atmosphere to densify it gradually. Actually, the direct exposure of an ecosystem to ultraviolet rays would be counterproductive for the carbon dioxide capture, biomass formation and ground gas generation. Precisely, the dome functions to protect an ecosystem from cold and ultraviolet radiation, as well as maintaining its inner pressure.
Now, the dome would be an important heat trap and a thermal insulator. Making the earlier cell analogy, the dome is like a biological membrane that drives the local ecosystem to thermodynamic disequilibrium. That disequilibrium would allow life to develop.
AM: Would high local concentrations of greenhouse gases (like methane, carbon dioxide or CFCs) be locally toxic before having any effects globally?
OPD: Life can adapt to conditions that are toxic for us; an elevated carbon dioxide concentration can be beneficial for plants, and even increase their production, or, as with methane, there are some methanogenic organisms that require this gas for their subsistence.
Such gases are appropriate for raising the global temperature; on the other hand, carbon dioxide is the most appropriate gas for plant life. The aim is to reproduce evolutionary patterns leading to a gradual adaptation of these organisms to a new environment, and the adaptation of the environment to these organism.
AM: Global terraforming on Mars has time ranges that vary between a century to even long times. Are there ways to estimate whether local efforts might accelerate habitability, using the oasis model you suggest?
OPD: That will depend on the plants’ photosynthetic efficiency and their capability to adapt themselves to the environment while adapting the environment. However, we can consider two appraisals: one local and one global.
In a more explicit way, those appraisals can be first measured on each Minimal Unit of Terraforming through its photosynthetic efficiency, oxygenation speed, carbon dioxide capture and degassing of the dome’s surface. This rate would depend on the solar incidence and the greenhouse effect. At a global level, the speed of the planet’s remodelling would depend on how many Minimal Units could be installed all over the Martian surface. That is to say, if there exist more Minimal Units of Terraforming, the planet’s transformation would be completed faster.
I’d like to clarify something I think is important at this point. The major achievement would be to turn Mars into a green planet before humans could inhabit it in the way we do on Earth today. It would be extraordinary to see how plant life responds, first inside the Minimal Unit of Terraforming and then, when those machines had finished their cycle and life emerges as an explosion to the exterior, to see the unstoppable speciation that would take place, since life would respond to the environment and the environment would respond to life.
And so, we may watch trees, such as pines that on Earth have a large and straight timber. On Mars we may have a more pliable species, one strong enough to resist low temperatures and blowing winds. As photosynthetic machines, the pines would be fulfilling their role as planetary transformers, keeping water, minerals and carbon dioxide for the accumulation of biomass.
AM: What future plans do you have for the research?
OPD: I want to initiate partial simulations of the Martian conditions. This is needed to probe and improve the operation of the Minimal Unit of Terraforming, as well as the physiological response of plants in such conditions. In other words, rehearsals.
This is a multidisciplinary and inter-institutional investigation, so the participation of engineers, biologists and genetic specialists will be necessary as well as other scientific organizations interested in the subject. I must say this is just the first attempt; it is a theory of what could be done and one that we could try on our own planet, for instance, by fighting against the aggressive desert spreading, by rehabilitating grounds and creating obstacles to stop its gradual advance.
Original Source: Astrobiology Magazine
Here’s an article about a similar project. Remember Biosphere 2?