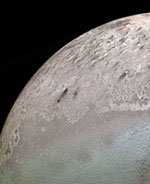
Image credit: NASA
We are doomed. One day the Earth will be a burnt cinder orbiting a swollen red star.
This is the ultimate fate of any planet living close to a main sequence star like our sun. Main sequence stars run on hydrogen, and when this fuel runs out, they switch over to helium and become a red giant. While the sun’s transition into a red giant is sad news for Earth, the icy planets in the most distant regions of our solar system will bask in the sun’s warmth for the first time.
The sun has been slowly but steadily growing brighter and hotter over the course of its lifetime. When the sun becomes a red giant in about 4 billion years, our familiar yellow sun will turn a vivid red, as it mainly emits the lower frequency energy of infrared and visible red light. It will grow thousands of times brighter and yet have a cooler surface temperature, and its atmosphere will expand, slowly engulfing Mercury, Venus and possibly even the Earth.
While the sun’s atmosphere is predicted to reach Earth’s orbit of 1 AU, red giants tend to lose a lot of mass, and this wave of expelled gases could push Earth just out of range. But whether the Earth is consumed or merely singed, all life on Earth will have passed into oblivion.
Yet the conditions that make life possible could appear elsewhere in the solar system, according to a paper published in the journal Astrobiology by S. Alan Stern, Director of the Southwest Research Institute’s Department of Space Studies in Boulder, Colorado. He says that planets located 10 to 50 AU will be in the red giant sun’s habitable zone. The habitable zone of a solar system is the region where water can remain in a liquid state.
The habitable zone will shift gradually through the 10 to 50 AU region as the sun grows brighter and brighter, evolving through its red giant phase. Saturn, Uranus, Neptune and Pluto all lie within 10 to 50 AU, as do their icy moons and the Kuiper Belt Objects. But not all these worlds will have an equal chance at life.
The prospects for habitability on the gaseous planets Saturn, Neptune and Uranus may not be affected all that much by the red giant transition. Astronomers have discovered gaseous planets orbiting very close to their parent star in other solar systems, and these “hot Jupiters” seem to hold onto their gaseous atmospheres despite their proximity to the intense radiation. Life as we know it is not likely to appear on gaseous planets.
Stern thinks Neptune’s moon Triton, Pluto and its moon Charon, and the Kuiper Belt Objects will have the best chances for life. These bodies are rich in organic chemicals, and the heat of the red giant sun will melt their icy surfaces into oceans.
“When the sun is a red giant, the ice worlds of our solar system will melt and become ocean oases for tens to several hundreds of millions of years,” says Stern. “Our solar system will then harbor not one world with surface oceans, as it does now, but hundreds, for all of the icy moons of the giant planets, and the icy dwarf planets of the Kuiper Belt will also bear oceans then. Because temperature on Pluto will not be very different then, than Miami Beach’s temperature now, I like to call these worlds ‘warm Plutos,’ in analogy to the plethora of hot Jupiters found orbiting sun-like stars in recent years.”
The influence of the sun is not the whole story, however – the characteristics of a planetary body go a long way toward determining habitability. Such characteristics include a planet’s internal activity, the reflectivity, or “albedo” of a planet, and the thickness and composition of the atmosphere. Even if a planet has all the elements that favor habitability, life will not necessarily appear.
“We don’t know what is needed to start life,” says Don Brownlee, an astronomer with the University of Washington in Seattle and co-author of the book, “The Life and Death of Planet Earth.” Brownlee says that if warm wet interiors and organic materials are all that’s needed, then Pluto, Triton, and the Kuiper Belt Objects could harbor life.
“As a word of caution, however, the interiors of asteroids that produced the carbonaceous chondrite meteorites were warm and wet for perhaps millions of years in the early history of the solar system,” says Brownlee. “These bodies are extremely rich in both water and organic materials, and yet there is no compelling evidence that any asteroidal meteorite ever had living things in it.”
A planetary body’s orbit also will affect its chances for life. Pluto, for instance, doesn’t have a nice, regular orbit like the Earth. The orbit of Pluto is comparatively eccentric, varying in distance from the sun. From January 1979 through February 1999, Pluto was closer to the sun than Neptune, and in a hundred years, it’ll be almost twice as far out as Neptune. This type of orbit will cause Pluto to undergo extreme heating alternating with extreme cooling.
Triton’s orbit, too, is peculiar. Triton is the only large moon to orbit backwards, or “retrograde.” Triton may have this unusual orbit because it formed as a Kuiper Belt Object and then was captured by Neptune’s gravity. It’s an unstable alliance, since the retrograde orbit creates tidal interactions with Neptune. Scientists predict that someday Triton will either crash into Neptune, or break up into tiny pieces and form a ring around the planet.
“The timescale for the tidal decay of Triton’s orbit is uncertain, so it could be around, or it might have already crashed by the time the sun goes red giant,” says Stern. “If Triton is around, it’ll probably end up looking like the same kind of organic-rich ocean world as Pluto.”
The sun will burn as a red giant for about 250 million years, but is that enough time for life to get a foothold? During most of the red giant lifetime, the sun will be only 30 times brighter than its current state. Toward the end of the red giant phase the sun will grow more than 1,000 times brighter, and occasionally release pulses of energy reaching 6,000 times current brightness. But this period of intense brightness will last for a few million years, or tens of millions of years at most.
The brevity of the red giant’s brightest phases suggests to Brownlee that Pluto doesn’t hold much promise for life. Because of Pluto’s average orbit of 40 AU, the sun would have to be 1,600 times brighter for Pluto to get the same solar radiation we currently get on Earth.
“The sun will reach this brightness, but only for a very brief period of time – only a million years or so,” says Brownlee. “The surface and atmosphere of Pluto will be ‘improved’ from our point of view, but it won’t be a nice place for any significant period of time”.
After the red giant phase, the sun will become fainter, and will shrink to the size of the Earth, becoming a white dwarf. The distant planets that basked in the light of the red giant will become frozen ice worlds once again.
So if life is to appear in a red giant system, it will need a quick start. Life on Earth is thought to have originated 3.8 billion years ago, some 800 million years after our planet was born. But that is probably because the planets in the inner solar system experienced 800 million years of heavy asteroid bombardment. Even if life had gotten started immediately, the early rain of asteroids would’ve wiped the Earth clean of that life.
Brownlee says a new era of bombardment could begin for the outer planets, because the red giant sun could disturb the vast number of comets in the Kuiper Belt.
“When the red giant sun is 1,000 times brighter, it loses almost half of its mass to space,” says Brownlee. “This causes orbiting bodies to move outward. Gas loss and other effects might destabilize the Kuiper Belt and create another period of interesting bombardment.”
But Stern says that planets made habitable by a red giant sun won’t be bombarded as often as the early Earth was, because the ancient asteroid belt had much more material than the Kuiper Belt has today.
In addition, the outer planets won’t experience the same ultraviolet (UV) levels that Earth has had to endure, since red giants have very low UV radiation. The higher intensity UV of a main sequence star can be damaging to the delicate proteins and RNA strands needed for life’s origin. Life on Earth could only originate underwater, in depths protected from this light intensity. Life on Earth is therefore inextricably linked to liquid water. But who knows what sort of life might originate on planets that have no need for UV shielding?
Stern thinks we should look for evidence of life on Pluto-like worlds orbiting around red giants today. We currently know of 100 million solar-type stars in the Milky Way galaxy that burn as red giants, and Stern says that all of these systems could have habitable planets within 10 to 50 AU. “It would be a good test of the time required to create life on warm, water-rich worlds,” he says.
“The idea of organic-rich distant bodies getting baked by a red giant star is an intriguing one, and could provide very interesting if short-lived habitats for life,” adds Brownlee. “But I am glad that our sun has a good margin of time left.”
What’s Next
While much of what we know about the outer solar system is based on distant measurements made from Earth-based telescopes, on January 2, 2004, scientists caught a close-up glimpse of a Kuiper Belt Object. The Stardust spacecraft passed within 136 kilometers of comet Wild2, an enormous snowball that spent most of its 4.6 billion-year lifetime orbiting in the Kuiper Belt. Wild2 now orbits mostly inside the orbit of Jupiter. Brownlee, who is the Principle Investigator for the Stardust mission, says that the Stardust images show fantastic surface details of a body shaped both by its ancient and recent history. Stardust images show gas and dust jets shooting off the comet, as Wild2 rapidly disintegrates in the strong solar heat of the inner solar system.
To learn more about the outer solar system, we’ll need to send a spacecraft out there to investigate. In 2001, NASA selected the New Horizons mission for just such a purpose.
Stern, who is the Principal Investigator for the New Horizons mission, reports that the spacecraft assembly is scheduled to begin this summer. The spacecraft is due to launch in January 2006, and arrive at Pluto the summer of 2015.
The New Horizons mission will allow scientists to study the geology of Pluto and Charon, map their surfaces, and take their temperatures. Pluto’s atmosphere also will be studied in detail. In addition, the spacecraft will visit the icy bodies in the Kuiper Belt in order to make similar measurements.
Original Source: Astrobiology Magazine