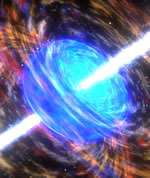
Image credit: NASA
Astronomers believe that gamma-ray bursts, the most powerful explosions in the Universe, may be generating ultrahigh-energy cosmic rays, the most energetic particles in the Universe. These cosmic rays have baffled astronomers because they’re moving faster than if they were thrown out of a supernova. Evidence gathered by NASA’s de-orbited Compton Gamma-Ray Observatory showed that in one instance of a gamma ray burst, these high-energy particles dominated the area giving a connection between them, but this is hardly enough evidence to say they’re conclusively linked.
The most powerful explosions in the universe, gamma-ray bursts, may generate the most energetic particles in the universe, known as the ultrahigh-energy cosmic rays (UHECRs), according to a new analysis of observations from NASA’s Compton Gamma-Ray Observatory.
Researchers report in the August 14 edition of Nature of a newly identified pattern in the light from these enigmatic bursts that could be explained by protons moving within a hair’s breadth of light speed.
These protons, like shrapnel from an explosion, could be UHECRs. Such cosmic rays are rare and constitute an enduring mystery in astrophysics, seemingly defying physical explanation, for they are simply far too energetic to have been generated by well-known mechanisms such as supernova explosions.
“Cosmic rays ‘forget’ where they come from because, unlike light, they are whipped about in space by magnetic fields,” said lead author Maria Magdalena Gonzalez of the Los Alamos National Laboratory in New Mexico and graduate student at the University of Wisconsin. “This result is an exciting chance to possibly see evidence of them being produced at their source.”
Gamma-ray bursts — a mystery scientists are finally beginning to unravel — can shine as brilliantly as a million trillion suns, and many may be from an unusually powerful type of exploding star. The bursts are common yet random and fleeting, lasting only seconds.
Cosmic rays are atomic particles (for example, electrons, protons or neutrinos) moving close to light speed. Lower-energy cosmic rays bombard the Earth constantly, propelled by solar flares and typical star explosions. UHECRs, with each atomic particle carrying the energy of a baseball thrown in the Major Leagues, are a hundred-million times more energetic than the particles produced in the largest human-made particle accelerators.
Scientists say the UHECRs must be generated relatively close to the Earth, for any particle traveling farther than 100 million light years would lose some of its energy by the time it reached us. Yet no local source of ordinary cosmic rays seems powerful enough to generate a UHECR.
The Gonzalez-led paper focuses not specifically on UHECR production but rather a new pattern of light seen in a gamma-ray burst. Digging deep into the Compton Observatory archives (the mission ended in 2000), the group found that a gamma-ray burst from 1994, named GRB941017, appears different from the other 2,700-some bursts recorded by this spacecraft. This burst was located in the direction of the constellation Sagitta, the Arrow, likely ten billion light years away.
What scientists call gamma rays are photons (light particles) covering a wide range of energies, in fact, over a million times wider than the energies our eyes register as the colors in a rainbow. Gonzalez’s group looked at the higher-energy gamma-ray photons. The scientists found that these types of photons dominated the burst: They were at least three times more powerful on average than the lower-energy component yet, surprisingly, thousands of times more powerful after about 100 seconds.
That is, while the flow of lower-energy photons hitting the satellite’s detectors began to ease, the flow of higher-energy photons remained steady. The finding is inconsistent with the popular “synchrotron shock model” describing most bursts. So what could explain this enrichment of higher-energy photons?
“One explanation is that ultrahigh-energy cosmic rays are responsible, but exactly how they create the gamma rays with the energy patterns we saw needs a lot of calculating,” said Dr. Brenda Dingus of LANL, a co-author on the paper. “We’ll be keeping some theorists busy trying to figure this out.”
A delayed injection of ultrahigh-energy electrons provides another way to explain the unexpectedly large high-energy gamma-ray flow observed in GRB 941017. But this explanation would require a revision of the standard burst model, said co-author Dr. Charles Dermer, a theoretical astrophysicist at the U.S. Naval Research Laboratory in Washington. “In either case, this result reveals a new process occurring in gamma-ray bursts,” he said.
Gamma-ray bursts have not been detected originating within 100 million light years from Earth, but through the eons these types of explosions may have occurred locally. If so, Dingus said, the mechanism her group saw in GRB 941017 could have been duplicated close to home, close enough to supply the UHECRs we see today.
Other bursts in the Compton Observatory archive may have exhibited a similar pattern, but the data are not conclusive. NASA’s Gamma-ray Large Area Space Telescope (GLAST), scheduled for launch in 2006, will have detectors powerful enough to resolve higher-energy gamma-ray photons and solve this mystery.
Co-authors on the Nature report also include Ph.D. graduate student Yuki Kaneko, Dr. Robert Preece, and Dr. Michael Briggs of the University of Alabama in Huntsville. This research was funded by NASA and the Office of Naval Research.
UHECRs are observed when they crash into our atmosphere, as is illustrated in the figure. The energy from the collision produces an air shower of billions of subatomic particles and flashes of ultraviolet light, which are detected by special instruments.
The National Science Foundation and international collaborators have sponsored instruments on the ground, such as the High Resolution Fly’s Eye in Utah (http://www.cosmic-ray.org/learn.html) and the Auger Observatory in Argentina (http://www.auger.org/). In addition, NASA is working with the European Space Agency to place the Extreme Universe Space Observatory (http://aquila.lbl.gov/EUSO/) on the International Space Station. The proposed OWL mission would, from orbit, look downward towards air showers, viewing a region as large as Texas.
These scientists record the flashes and take a census of the subatomic shrapnel, working backward to calculate how much energy a single particle needs to make the atmospheric cascade. They arrive at a shocking figure of 10^20 electron volts (eV) or more. (For comparison, the energy in a particle of yellow light is 2 eV, and the electrons in your television tube are in the thousand electron volt energy range.)
These ultrahigh-energy particles experience the bizarre effects predicted by Einstein’s theory of special relativity. If we could observe them coming from a remote corner of the cosmos, say a hundred million light years away, we’d have to be patient — it will take a hundred million years to complete the journey. However, if we could travel with the particles, the trip is over in less than a day due to the dilation of time of rapidly moving objects as measured by an observer.
The highest energy cosmic rays cannot even reach us if produced from distant sources, because they collide and lose energy with the cosmic microwave photons left over from the big bang. Sources of these cosmic rays must be found relatively close to us, at a distance of several hundred million light years. Stars that explode as gamma-ray bursts are found within this distance, so intensive observational efforts are underway to find gamma-ray burst remnants distinguished by radiation halos made by the cosmic rays.
Few kinds of celestial objects possess the extreme conditions required to blast particles to UHECR speeds. If gamma-ray bursts produce UHECRs, they probably do so by accelerating particles in jets of matter ejected from the explosion at close to the speed of light. Gamma-ray bursts have the power to accelerate UHECRs, but the gamma-ray bursts observed so far have been remote, billions of light years away. This doesn’t mean they can’t happen nearby, within the UHECR cutoff distance.
A leading contender for long-lived kinds of gamma-ray bursts like GRB941017 is the supernova/collapsar model. Supernovae happen when a star many times more massive than the Sun exhausts its fuel, causing its core to collapse under its own gravity while its outer layers are blown off in an immense thermonuclear explosion. Collapsars are a special type of supernova where the core is so massive it collapses into a black hole, an object so dense that nothing, not even light, can escape its gravity within the black hole’s event horizon. However, observations indicate black holes are sloppy eaters, ejecting material that passes near, but does not cross, their event horizons.
In a collapsar, the star’s core forms a disk of material around the newly formed black hole, like water swirling around a drain. The black hole consumes most of the disk, but some matter is blasted in jets from the poles of the black hole. The jets tear through the collapsing star at close to the speed of light, and then punch through gas surrounding the doomed star. As the jets crash into the interstellar medium, they create shock waves and slow down. Internal shocks also form in the jets as their leading edges slow and are slammed from behind by a stream of high-speed matter. The shocks accelerate particles that generate gamma rays; they could also accelerate particles to UHECR speeds, according to the team.
“It’s like bouncing a ping pong ball between a paddle and a table,” said Dingus. “As you move the paddle closer to the table, the ball bounces faster and faster. In a gamma-ray burst, the paddle and the table are shells ejected in the jet. Turbulent magnetic fields force the particles to ricochet between the shells, accelerating them to almost the speed of light before they break free as UHECRs.”
Detection of neutrinos from gamma-ray bursts would clinch the case for cosmic ray acceleration by gamma-ray bursts. Neutrinos are elusive particles made when high-energy protons collide with photons. Neutrinos have no electrical charge, so still point back to the direction of their source.
The National Science Foundation is currently building IceCube (http://icecube.wisc.edu/), a cubic kilometer detector located in the ice under the South Pole, to search for neutrino emission from gamma-ray bursts. However, the characteristics of nature’s highest-energy particle accelerators remain an enduring mystery, though acceleration by the exploding stars that make gamma-ray bursts has been in favor ever since Mario Vietri (Universita di Roma) and Eli Waxman (Weizmann Institute) proposed it in 1995.
The team believes that while other explanations are possible for this observation, the result is consistent with UHECR acceleration in gamma-ray bursts. They saw both low-energy and high-energy gamma rays in the GRB941017 explosion. The low-energy gamma rays are what scientists expect from high-speed electrons being deflected by intense magnetic fields, while the high-energy rays are what’s expected if some of the UHECRs produced in the burst crash into other photons, creating a shower of particles, some of which flash to produce the high-energy gamma rays when they decay.
The timing of the gamma-ray emission is also significant. The low-energy gamma rays faded away relatively quickly, while the high-energy gamma rays lingered. This makes sense if two different classes of particles – electrons and the protons of the UHECRs – are responsible for the different gamma rays. “It’s much easier for electrons than protons to radiate their energy. Therefore, the emission of low-energy gamma rays from electrons would be shorter than the high-energy gamma rays from the protons,” said Dingus.
The Compton Gamma Ray Observatory was the second of NASA’s Great Observatories and the gamma-ray equivalent to the Hubble Space Telescope and the Chandra X-ray Observatory. Compton was launched aboard the Space Shuttle Atlantis in April 1991, and at 17 tons, was the largest astrophysical payload ever flown at that time. At the end of its pioneering mission, Compton was deorbited and re-entered the Earth’s atmosphere on June 4, 2000.
Original Source: NASA News Release