When it comes to objects and force, Isaac Newton’s Three Laws of Motion are pretty straightforward. Apply force to an object in a specific direction, and the object will move in that direction. And unless there’s something acting against it (like gravity or air pressure) it will keep moving in that direction until something stops it. But when it comes to “negative mass”, the exact opposite is true.
As the name would suggest, the term refers to matter whose mass is opposite that of normal matter. Until a few years ago, negative mass was predominantly a theoretical concept and had only been observed in very specific settings. But according to a recent study by an international team of researchers, they managed to create a fluid with a “negative effective mass” under laboratory conditions for the first time .
To put it in the simplest terms, matter can have a negative mass in the same way that a particle can have a negative charge. When it comes to the Universe that we know and study on a regular basis, one could say that we have encountered only the positive form of mass. In fact, one could say that it is the same situation with matter and antimatter. Theoretical physics tells us both exist, but we only see the one on a regular basis.
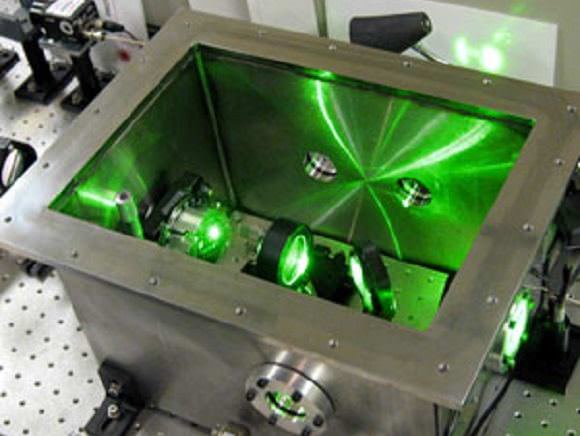
As Dr. Michael McNeil Forbes – a Professor at Washington State University, a Fellow at the Institute for Nuclear Theory, and a co-author on the study – explained in a WSU press release:
“That’s what most things that we’re used to do. With negative mass, if you push something, it accelerates toward you. Once you push, it accelerates backwards. It looks like the rubidium hits an invisible wall.”
According to the team’s study, which was recently published in the Physical Review Letters (under the title “Negative-Mass Hydrodynamics in a Spin-Orbit–Coupled Bose-Einstein Condensate“), a negative effective mass can be created by altering the spin-orbit coupling of atoms. Led by Peter Engels – a professor of physics and astronomy at Washington State University – this consisted of using lasers to control the behavior of rubidium atoms.
They began by using a single laser to keep rubidium atoms in a bowl measuring less than 100 microns across. This had the effect of slowing the atoms down and cooling them to just a few degrees above absolute zero, which resulted in the rubidium becoming a Bose-Einstein condensate. Named after Satyendra Nath Bose and Albert Einstein (who predicted how their atoms would behave) these types of condensates behaves like a superfluid.
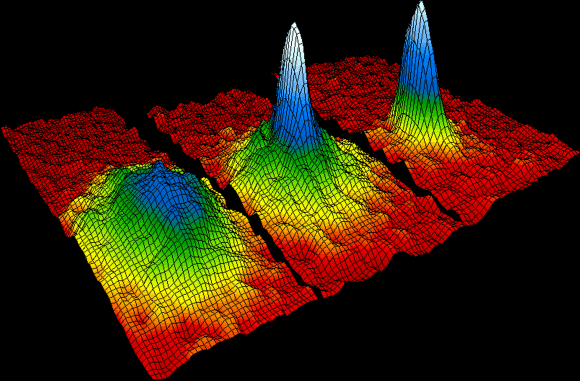
Basically, this means that their particles move very slowly and behave like waves, but without losing any energy. A second set of lasers was then applied to move the atoms back and forth, effectively changing the way they spin. Prior to the change in their spins, the superfluid had regular mass and breaking the bowl would result in them pushing out and expanding away from their center of mass.
But after the application of the second laser, the rubidium rushed out and accelerated in the opposite direction – consistent with how a negative mass would. This represented a break with previous laboratory experiments, where researchers were unable to get atoms to behave in a way that was consistent with negative mass. But as Forbes explained, the WSU experiment avoided some of the underlying defects encountered by these experiments:
“What’s a first here is the exquisite control we have over the nature of this negative mass, without any other complications. It provides another environment to study a fundamental phenomenon that is very peculiar.”
And while news of this experiment has been met with fanfare and claims to the effect that the researchers had “rewritten the laws of physics”, it is important to emphasize that this research has created a “negative effective mass” – which is fundamentally different from a negative mass.
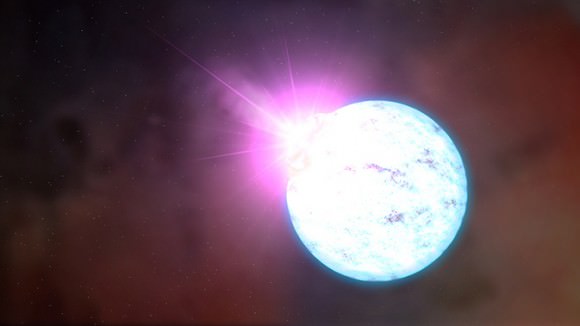
Credit: NASA/Goddard Space Flight Center
As Sabine Hossenfelder, a Research Fellow at the Frankfurt Institute for Advanced Studies, wrote on her website Backreaction in response to the news:
“Physicists use the preamble ‘effective’ to indicate something that is not fundamental but emergent, and the exact definition of such a term is often a matter of convention. The ‘effective radius’ of a galaxy, for example, is not its radius. The ‘effective nuclear charge’ is not the charge of the nucleus. And the ‘effective negative mass’ – you guessed it – is not a negative mass. The effective mass is merely a handy mathematical quantity to describe the condensate’s behavior.”
In other words, the researchers were able to get atoms to behave as a negative mass, rather than creating one. Nevertheless, their experiment demonstrates the level of control researchers now have when conducting quantum experiments, and also serves to clarify how negative mass behaves in other systems. Basically, physicists can use the results of these kinds of experiments to probe the mysteries of the Universe where experimentation is impossible.
These include what goes on inside neutron stars or what transpires beneath the veil of a event horizon. Perhaps they could even shed some light on questions relating to dark energy.
Further Reading: Physical Review Letters, WSU