Gravity is a pretty awesome fundamental force. If it wasn’t for the Earth’s comfortable 1 g, which causes objects to fall towards the Earth at a speed of 9.8 m/s², we’d all float off into space. And without it, all us terrestrial species would slowly wither and die as our muscles degenerated, our bones became brittle and weak, and our organs ceased to function properly.
So one can say without exaggerations that gravity is not only a fact of life here on Earth, but a prerequisite for it. However, since human beings seem intent on getting off this rock – escaping the “surly bonds of Earth”, as it were – understanding Earth’s gravity and what it takes to escape it is necessary. So just how strong is Earth’s gravity?
Definition:
To break it down, gravity is a natural phenomena in which all things that possess mass are brought towards one another – i.e. asteroids, planets, stars, galaxies, super clusters, etc. The more mass an object has, the more gravity it will exert on objects around it. The gravitational force of an object is also dependent on distance – i.e. the amount it exerts on an object decreases with increased distance.
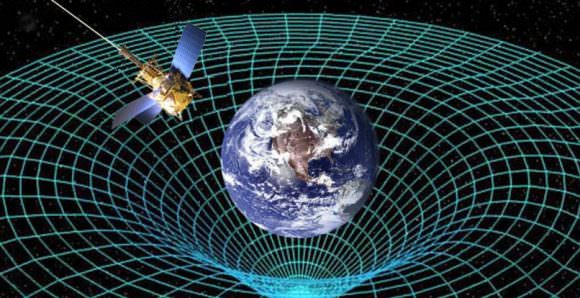
Gravity is also one of the four fundamental forces which govern all interactions in nature (along with weak nuclear force, strong nuclear force, and electromagnetism). Of these forces, gravity is the weakest, being approximately 1038 times weaker than the strong nuclear force, 1036 times weaker than the electromagnetic force and 1029 times weaker than the weak nuclear force.
As a consequence, gravity has a negligible influence on matter at the smallest of scales (i.e. subatomic particles). However, at the macroscopic level – that of planets, stars, galaxies, etc. – gravity is the dominant force affecting the interactions of matter. It causes the formation, shape and trajectory of astronomical bodies, and governs astronomical behavior. It also played a major role in the evolution of the early Universe.
It was responsible for matter clumping together to form clouds of gas that underwent gravitational collapse, forming the first stars – which were then drawn together to form the first galaxies. And within individual star systems, it caused dust and gas to coalesce to form the planets. It also governs the orbits of the planets around stars, of moons around planets, the rotation of stars around their galaxy’s center, and the merging of galaxies.
Universal Gravitation and Relativity:
Since energy and mass are equivalent, all forms of energy, including light, also cause gravitation and are under the influence of it. This is consistent with Einstein’s General Theory of Relativity, which remains the best means of describing gravity’s behavior. According to this theory, gravity is not a force, but a consequence of the curvature of spacetime caused by the uneven distribution of mass/energy.
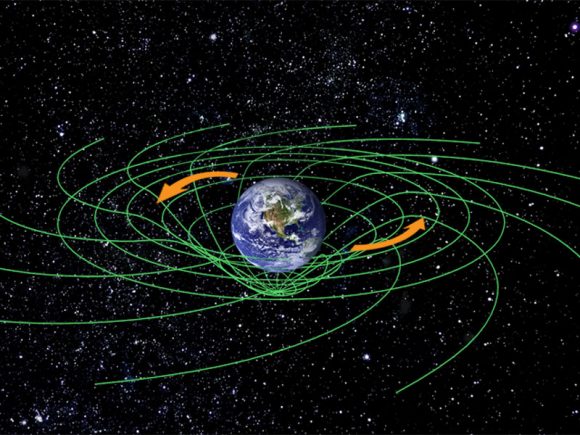
The most extreme example of this curvature of spacetime is a black hole, from which nothing can escape. Black holes are usually the product of a supermassive star that has gone supernova, leaving behind a white dwarf remnant that has so much mass, it’s escape velocity is greater than the speed of light. An increase in gravity also results in gravitational time dilation, where the passage of time occurs more slowly.
For most applications though, gravity is best explained by Newton’s Law of Universal Gravitation, which states that gravity exists as an attraction between two bodies. The strength of this attraction can calculated mathematically, where the attractive force is directly proportional to the product of their masses and inversely proportional to the square of the distance between them.
Earth’s Gravity:
On Earth, gravity gives weight to physical objects and causes the ocean tides. The force of Earth’s gravity is the result of the planets mass and density – 5.97237 × 1024 kg (1.31668×1025 lbs) and 5.514 g/cm3, respectively. This results in Earth having a gravitational strength of 9.8 m/s² close to the surface (also known as 1 g), which naturally decreases the farther away one is from the surface.
In addition, the force of gravity on Earth actually changes depending on where you’re standing on it. The first reason is because the Earth is rotating. This means that the gravity of Earth at the equator is 9.789 m/s2, while the force of gravity at the poles is 9.832 m/s2. In other words, you weigh more at the poles than you do at the equator because of this centripetal force, but only slightly more.
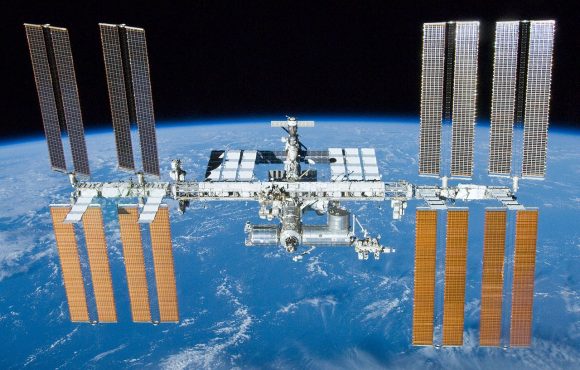
Finally, the force of gravity can change depending on what’s under the Earth beneath you. Higher concentrations of mass, like high-density rocks or minerals can change the force of gravity that you feel. But of course, this amount is too slight to be noticeable. NASA missions have mapped the Earth’s gravity field with incredible accuracy, showing variations in its strength, depending on location.
Gravity also decreases with altitude, since you’re further away from the Earth’s center. The decrease in force from climbing to the top of a mountain is pretty minimal (0.28% less gravity at the top of Mount Everest), but if you’re high enough to reach the International Space Station (ISS), you would experience 90% of the force of gravity you’d feel on the surface.
However, since the station is in a state of free fall (and also in the vacuum of space) objects and astronauts aboard the ISS are capable of floating around. Basically, since everything aboard the station is falling at the same rate towards the Earth, those aboard the ISS have the feeling of being weightless – even though they still weight about 90% of what they would on Earth’s surface.
Earth’s gravity is also responsible for our planet having an “escape velocity” of 11.186 km/s (or 6.951 mi/s). Essentially, this means that a rocket needs to achieve this speed before it can hope to break free of Earth’s gravity and reach space. And with most rocket launches, the majority of their thrust is dedicated to this task alone.
Because of the difference between Earth’s gravity and the gravitational force on other bodies – like the Moon (1.62 m/s²; 0.1654 g) and Mars (3.711 m/s²; 0.376 g) – scientists are uncertain what the effects would be to astronauts who went on long-term missions to these bodies.
While studies have shown that long-duration missions in microgravity (i.e. on the ISS) have a detrimental effect on astronaut health (including loss of bone density, muscle degeneration, damage to organs and to eyesight) no studies have been conducted regarding the effects of lower-gravity environments. But given the multiple proposals made to return to the Moon, and NASA’s proposed “Journey to Mars“, that information should be forthcoming!
As terrestrial beings, we humans are both blessed and cursed by the force of Earth’s gravity. On the one hand, it makes getting into space rather difficult and expensive. On the other, it ensures our health, since our species is the product of billions of years of species evolution that took place in a 1 g environment.
If we ever hope to become a truly space-faring and interplanetary species, we better figure out how we’re going to deal with microgravity and lower-gravity. Otherwise, none of us are likely to get off-world for very long!
We have written many articles about the Earth for Universe Today. Here’s Where Does Gravity Come From?, Who Discovered Gravity?, Why is the Earth Round?, Why Doesn’t the Sun Steal the Moon?, Could We Make Artificial Gravity?, and The “Potsdam Gravity Potato” Shows Variations in Earth’s Gravity.
Want more resources on the Earth? Here’s a link to NASA’s Human Spaceflight page, and here’s NASA’s Visible Earth.
We have also recorded an episode of Astronomy Cast about Earth, as part of our tour through the Solar System – Episode 51: Earth, and Episode 318: Escape Velocity.
Sources: