Will Gater from the BBC’s Sky at Night Magazine shared this great video with us that shows some of the celestial sights that are you can see from your own backyard during the summer months. The video includes locator charts for all of the objects too, so you can see exactly where to find what you are looking for.
Cosmology in the Year 1 Trillion
[/caption]Much of what is known today about the birth of the cosmos comes from astronomical observations at high redshifts. Due to the accelerated expansion of the Universe, however, astronomers of the future will be unable to use the same methods. In a trillion years or so, our own Milky Way galaxy will have merged with the Andromeda galaxy, creating a new galaxy that has been quaintly termed “Milkomeda.” All of our other galactic neighbors will have long disappeared beyond our cosmological horizon. Even the CMB will have been stretched into invisibility. So how will future Milkomedans study cosmology? How will they figure out where the Universe came from?
According to a paper published by the Harvard-Smithsonan Center for Astrophysics, these astronomers will be able to decode the secrets of the cosmos by studying stellar runaways from their own galaxy: so-called hypervelocity stars (HVSs). HVSs originate in binary or triple-star systems that wander just a hair too close to their galaxy’s central supermassive black hole. Astronomers believe that one star from the system is captured by the black hole, while the others are sent careening out of the galaxy at colossally high speeds. HVS ejections occur relatively rarely (approximately once every 10,000-100,000 years) and should continue to occur for trillions of years, given the large density of stars in the galactic center.
So how would HVSs help future astronomers study the origins of the Universe? First, these scientists would have to locate an ejected star beyond the gravitational boundary of Milkomeda. Once beyond this boundary (after about 2 billion years of travel), the acceleration of a HVS could be attributed entirely to the Hubble flow. With advanced technology, future astronomers could use the Doppler shift of its spectral lines and thus deduce Einstein’s cosmological constant and the acceleration of the Universe at large. Next, scientists could use mathematical models of galaxy formation and collapse to determine the Universe’s mass density and age at the time that Milkomeda formed. From their knowledge of the galaxy’s age, they would be able to tell when the Big Bang occurred.
Guest Post: Newly Born: the Science of Astronomy
[/caption]
Editor’s Note: Astronomy journalist Govert Schilling has written a book that looks at the 100 most important discoveries since the invention of the telescope 400 years ago, called “Atlas of Astronomical Discoveries.” In Schilling’s distinct style, he takes the reader on an adventure through both space and time. Schilling has written this guest post for Universe Today:
Astronomy is a newborn science.
Yes, I know astronomers like to say it’s the oldest science in the world. In a sense, our distant ancestors who wondered about the lights and motions in the night sky were the first practitioners.
But look at it this way: until four centuries ago, we all had the same opportunities in the field. Or lack thereof. Two eyes and a brain – that has been the main instrumentation in astronomy for thousands of years. Not much, really.
Little wonder then that astronomy was in a pretty primitive state at the start of the seventeenth century. Granted, scientists had come to realize that the Sun occupied the center of the solar system, rather than the Earth. They had seen the occasional comet and Stella Nova, and they knew about the slow change in the orientation of the Earth’s axis.
But no one knew the distances to the planets, let alone to the stars. No one had the slightest clue about the true nature of the Sun or the Moon. Meteors were a mystery; planetary satellites and rings were unheard of, and to many, the Milky Way was just that – a cosmic river of milky clouds.
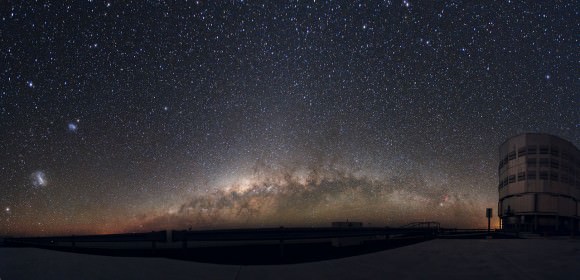
More importantly, no one realized that the Universe is in a constant state of flux, albeit at an extremely slow pace. That stars were once born and will eventually die. That the planets in our solar system are built from the ashes of an earlier generation of stars. That the Universe hasn’t always been there.
Most of the astronomical knowledge that we take for granted these days, was completely unknown four centuries ago. That’s why I say astronomy is a newborn science.
And the telescope was its midwife.

The invention of the telescope, probably around 1600 in the Netherlands, ushered in a whole new scientific era. It paved the way for hundreds of revolutionary discoveries and revealing insights. It brought astronomy to where it is now.
On the occasion of the International Year of Astronomy (2009), I decided to devote a book to the hundred most important astronomical discoveries since the invention of the telescope. Recently translated into English as Atlas of Astronomical Discoveries (Springer, 2011), it is a lavishly illustrated and beautifully designed history tour of the grandest science of all, chockfull with surprising details and personal anecdotes.
What I realized when writing the book was that the young science of astronomy went through a number of very distinct stages, just like a human being goes through childhood, puberty and adolescence before reaching full maturity.
In the seventeenth century, astronomers were like children in a newly opened candy store. Wherever they aimed their rather primitive telescopes, they beheld new discoveries, but this embarrassment of riches was also an undirected endeavour.
During the eighteenth century, the search became more systematic, with diligent observers surveying the skies and taking stock of everything that the telescope brought into view. This was no longer a first reconnaissance, but a real exploratory phase.
Then came the nineteenth century, with the advent of photography and spectroscopy, and the discovery of mysterious cosmic denizens like spiral nebulae, white dwarfs, and interstellar matter. Nature was trying to tell us something profound, and astronomy stood on the threshold of major theoretical breakthroughs that would explain this surprising variety of phenomena.
Finally, the twentieth century saw the emergence of an interconnected, all-encompassing view of cosmic evolution. We discovered the energy source of stars, the true nature of galaxies, the expansion of the Universe, and the humble position of our home planet, both in space and in time. Moreover, we finally understood that the atoms in our bodies were forged in the nuclear ovens of distant suns. That we are truly one with the Universe.
So has astronomy grown into a mature science? With the current generation of giant telescopes, the full exploration of the electromagnetic spectrum, and the advent of space science and computer technology, it’s tempting to answer this question with a resounding ‘yes’. Then again, ninety-six percent of the cosmos consists of mysterious dark matter and dark energy; we have no clue about the origin of our Universe, and no one knows whether or not life – let alone intelligence – is rare or abundant.
Personally, I feel that astronomy is still in its early years. And that’s exactly why it fires the imagination of so many people. The questions that astronomers try to answer are the same questions that a ten-year old would ask. The answers may be difficult, but the questions are simple, because the science is young. What’s it made of? How did it all start? Are we alone?
Certainly, I’d love to see a 2411 edition of Atlas of Astronomical Discoveries, highlighting the hundred most important discoveries and breakthroughs that astronomers made in the 21st, 22nd, 23rd and 24th century. But I’m afraid I wouldn’t understand most of the issues that would be described.
Frankly, I’m glad to live during the youth of my favorite science. After all, I’ve always been fond of the curiosity, energy, creativity and the sheer sense of wonder of children.
Please, astronomy, don’t grow up too soon.
Govert Schilling is an internationally acclaimed astronomy writer in the Netherlands. He is a contributing editor of Sky & Telescope, and his articles have appeared in Science, New Scientist and BBC Sky at Night Magazine. He wrote over fifty books on a wide variety of astronomical topics, some of which have been translated into English, including “Evolving Cosmos; Flash! The Hunt for the Biggest Explosions in the Universe,” TThe Hunt for Planet X,” and “Atlas of Astronomical Discoveries.” In 2007, the International Astronomical Union named asteroid (10986) Govert after him.
Testing the Spiral Density Wave Theory
[/caption]
Spiral galaxies are one of the most captivating structures in astronomy, yet their nature is still not fully understood. Astronomers currently have two categories of theories that can explain this structure, depending on the environment of the galaxy, but a new study, accepted for publication in the Astrophysical Journal, suggests that one of these theories may be largely wrong.
For galaxies with nearby companions, astronomers have suggested that tidal forces may draw out spiral structure. However, for isolated galaxies, another mechanism is required in which galaxies form these structures without intervention from a neighbor. A possible solution to this was first worked out in 1964 by Lin & Shu in which they suggested that the winding structure is merely an illusion. Instead, these arms weren’t moving structures, but areas of greater density which remained stationary as stars entered and exited them similar to how a traffic jam remains in position although the component cars travel in and out. This theory has been dubbed the Lin-Shu density wave theory and has been largely successful. Previous papers have reported a progression from cold, HI regions and dust on the inner portion of the spiral arms, that crash into this higher density region and trigger star formation, making hot O & B class stars that die before exiting the structure, leaving the lower mass stars to populate the remainder of the disk.
One of the main questions on this theory has been the longevity of the overdense region. According to Lin & Shu as well as many other astronomers, these structures are generally stable over long time periods. Others suggest that the density wave comes and goes in relatively short-lived, recurrent patterns. This would be similar to the turn signal on your car and the one in front of you at times seeming to synch up before getting out of phase again, only to line up again in a few minutes. In galaxies, the pattern would be composed of the individual orbits of the stars, which would periodically line up to create the spiral arms. Teasing out which of these was the case has been a challenge.
To do so, the new research, led by Kelly Foyle from McMaster University in Ontario, examined the progression of star formation as gas and dust entered the shock region produced by the Lin-Shu density wave. If the theory was correct, they should expect to find a progression in which they would first find cold HI gas and carbon monoxide, and then offsets of warm molecular hydrogen and 24 μm emission from stars forming in clouds, and finally, another offset of the UV emission of fully formed and unobscured stars.
The team examined 12 nearby spiral galaxies, including M 51, M 63, M 66, M 74, M 81, and M 95. These galaxies represented several variations of spiral galaxies such as grand design spirals, barred spirals, flocculent spirals and an interacting spiral.
When using a computer algorithm to examine each for offsets that would support the Lin-Shu theory, the team reported that they could not find a difference in location between the three different phases of star formation. This contradicts the previous studies (which were done “by eye” and thus subject to potential bias) and casts doubt on long lived spiral structure as predicted by the Lin-Shu theory. Instead, this finding is in agreement with the possibility of transient spiral arms that break apart and reform periodically.
Another option, one that salvages the density wave theory is that there are multiple “pattern speeds” producing more complex density waves and thus blurs the expected offsets. This possibility is supported by a 2009 study which mapped these speeds and found that several spiral galaxies are likely to exhibit such behavior. Lastly, the team notes that the technique itself may be flawed and underestimating the emission from each zone of star formation. To settle the question, astronomers will need to produce more refined models and explore the regions in greater detail and in more galaxies.
Beginner’s Guide to Astronomy – Refractor Telescopes
If you ask someone to describe or draw a telescope, nine times out of ten it will be a refractor.
The refractor telescope is quite possibly the most common or easily recognized telescope. It is a very simple design, which has been around for hundreds of years.
The history of the refractor is that it was first invented in the Netherlands in 1608, and is credited to 3 individuals; Hans Lippershey, Zacharias Janssen – spectacle-makers and Jacob Metius.
In 1609 Galileo Galilei heard about the refracting telescope and made his own design, publically announcing his invention and further developing it through extensive experimentation. Galileo’s friend Johannes Kepler further experimented with the design, introducing convex lenses at both ends, improving the operation of the telescope.
Many advances were made and the refracting telescope became the primary instrument for astronomical observations, but there was one problem; they were huge and some were many tens of feet long!
But now, after more than 400 years and — luckily — through advances in know-how and technology, the refractor has become much more powerful and compact than some of the behemoths in the early days.
Refractors or refracting telescopes employ a simple optical system comprising of a hollow tube with a large primary or “objective lens” at one end, which refracts light collected by the objective lens and bends light rays to make them converge at a focal point.
Light waves which enter at an angle converge on the focal plane. It is the combination of both which form an image that is further refracted and magnified by a secondary lens which is actually the eyepiece. Different eyepieces give different magnifications.
The larger the size of the objective or primary lens = more light gathered. So a 6 inch refractor gathers more light than a 2 inch one. This means more detail can be seen.
There are two main types of refractor telescopes: “Chromatic” – entry level and upwards with 2 lens elements and “Apochromatic” – premium, advanced and expert level telescopes with 3 or more very high quality lens elements with exotic mixes of materials.
Chromatic refractor telescopes are particularly good for observing bright objects such as the moon, planets and resolving things like double stars, but many astronomers who image deep sky and other objects use very high quality apochromatic refractors, due to their superior optics.
Refractor telescopes are very low maintenance due to being a sealed system and it is a simple case of setup and enjoy, without the fiddling lengthy setup times you may get with other telescopes.
Refractors give clean and crisp views due to the sealed nature, unlike other telescopes like Newtonians which are subject to cooling and air turbulence issues.
Due to their small size they are very portable and can also be used for terrestrial observations the same as binoculars, which are basically two refractors bolted together.
Coming Soon – Night Shining Noctilucent Clouds
[/caption]
Soon you may see an eerie spectacle on clear summer nights if you are located at latitudes between 50° and 70° north and south of the equator: Noctilucent Clouds.
These ghostly apparitions are a delight to see and are quite rare. It is incredibly difficult to predict exactly when they will appear, but we do know they should begin to appear soon.
The season for Noctilucent Clouds (Noctilucent = Latin for “Night Shining”) starts early June and continues into late July. They are seen just after dusk, or before dawn and an apparition can last around an hour.
These mysterious clouds, with their bizarre tenuous wispy shapes reminiscent of ripples in sand or the changing surface of a pool of water, spread like a glowing web across the northern sky. Colours can range from brilliant whites, with tinges of blue, pink and orange.
Formed by tiny ice crystals, they are the highest clouds in the Earth’s atmosphere, located in the mesosphere at altitudes of around 76 to 85 kilometers (47 to 53 miles) almost at the edge of space.
They are normally too faint to be seen, and are visible only when illuminated by sunlight from below the horizon, while the lower layers of the atmosphere are in the Earth’s shadow. Noctilucent clouds are not fully understood and are a recently discovered meteorological phenomenon, only being recorded for about 120 years.
Noctilucent clouds can only form under very restrictive conditions, and their occurrence can be used as a guide to changes in the upper atmosphere. Since their relatively recent classification, the occurrence of noctilucent clouds appears to be increasing in frequency, brightness and extent.
There is evidence that the relatively recent appearance of noctilucent clouds and their gradual increase, may be linked to climate change. Another recent theory is that some of these bright displays come from particulates and water vapour in the atmosphere left over from Space Shuttle launches.
How can you see them? Over the next couple of months look north during dusk and dawn and try and spot this mysterious and elusive phenomenon. They are best seen when the sun is between 6 and 16 degrees below the horizon, and seem to occur more frequently in the Northern hemisphere than the Southern.
Good luck!

Examining the Great Wall
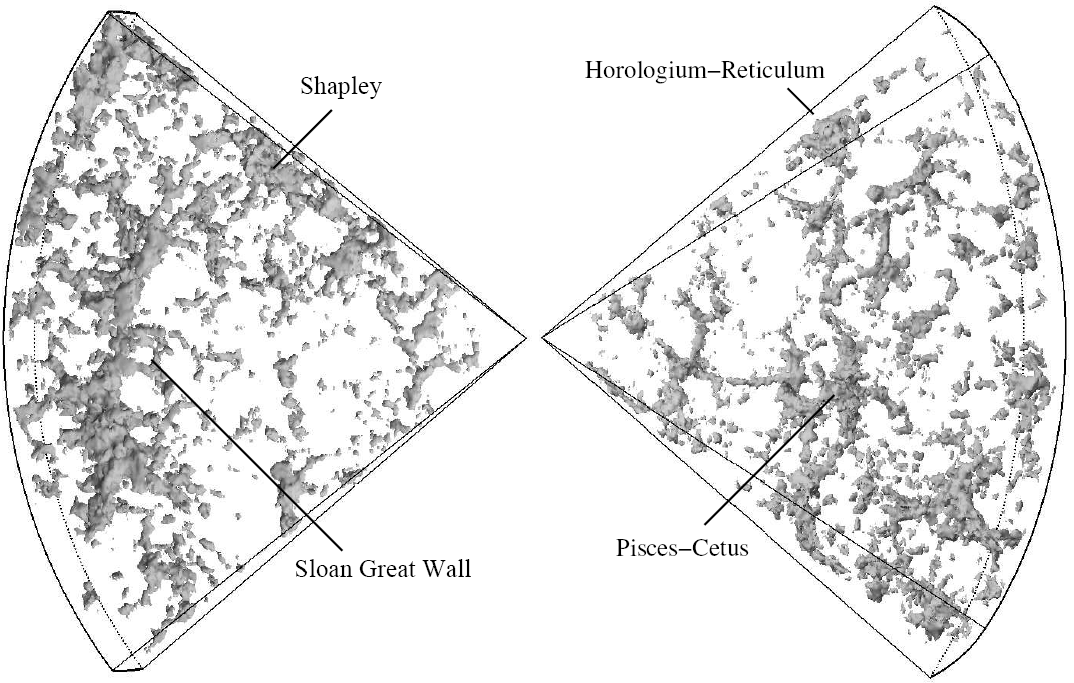
[/caption]
Structure exists on nearly all scales in the universe. Matter clumps under its own gravity into planets, stars, galaxies, clusters, and superclusters. Beyond even these in scale are the filaments and voids. The largest of these filaments is known as the Sloan Great Wall. This giant string of galaxies is 1.4 billion light years across making it the largest known structure in the universe. Yet surprisingly, the Great Wall has never been studied in detail. Superclusters within it have been examined, but the wall as a whole has only come into consideration in a new paper from a team led by astronomers at Tartu Observatory in Estonia.
The Sloan Great Wall was first discovered in 2003 from the Sloan Digital Sky Survey (SDSS). The survey mapped the position of hundreds of millions of galaxies revealing the large scale structure of the universe and uncovering the Great Wall.
Within it, the wall contains several interesting superclusters. The largest of these SCl 126 has been shown previously to be unusual compared to superclusters within other large scale structures. SCl 126 is described as having an exceptionally rich core of galaxies with tendrils of galaxies trailing away from it like an enormous “spider”. Typical superclusters have many smaller clusters connected by these threads. This pattern is exemplified by one of the other rich superclusters in the wall, SCl 111. If the wall is examined in only its densest portions, the tendrils extending away from these cores are quite simple, but as the team explored lower densities, sub filaments became apparent.
Another way the team examined the Great Wall was by looking at the arrangement of different types of galaxies. In particular, the team looked for Bright Red Galaxies (BRGs) and found that these galaxies are often found together in groups with at least five BRGs present. These galaxies were often the brightest of the galaxies within their own groups. As a whole, the groups with BRGs tended to have more galaxies which were more luminous, and have a greater variety of velocities. The team suggests that this increased velocity dispersion is a result of a higher rate of interactions among galaxies than in other clusters. This is especially true for SCl 126 where many galaxies are actively merging. Within SCl 126, these BRG groups were evenly distributed between the core and the outskirts while in SCl 111, these groups tended to congregate towards the high density regions. In both of these superclusters, spiral galaxies comprised about 1/3 of the BRGs.
The study of such properties will help astronomers to test cosmological models that predict galactic structure formation. The authors note that models have generally done a good job of being able to account for structures similar to SCl 111 and most other superclusters we have observed in the universe. However, they fall short in creating superclusters with the size, morphology and distribution of SCl 126. These formations arise from density fluctuations initially present during the Big Bang. As such, understanding the structures they formed will help astronomers to understand these perturbations in greater detail and, in turn, what physics would be necessary to achieve them. To help achieve this, the authors intend to continue mapping the morphology of the Sloan Great Wall as well as other superclusters to compare their features.
Clyde Tombaugh’s Ten Special Commandments for Planet Hunters
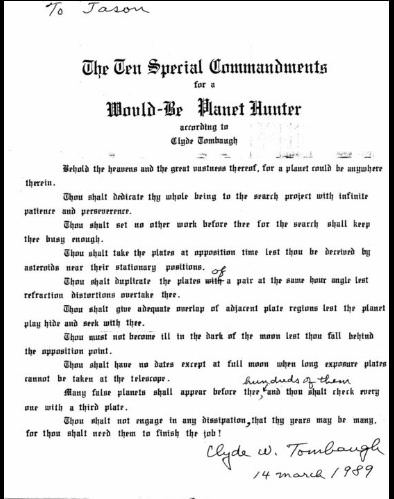
[/caption]
Back in 1989, amateur astronomer Toney Burkhart found out that Clyde Tombaugh was going to be giving a talk in San Francisco, just a short distance from Burkhart’s home. Trouble was, he found out only about 10 minutes before the presentation was going to start, so he rushed over and arrived just in time to hear Tombaugh’s talk, where he told amusing stories of how he found Pluto, and what he went through with night after night in a cold observatory taking photographs and comparing the glass plates, looking for a planet in the outer solar system. Then Tombaugh shared read his version of the Ten Commandments, called, “Ten Special Commandments for a Would-Be Planet Hunter.”
Afterward, the posters of the Commandments were being sold as a fund raising event.
“Clyde was going around the country to raise money for scholarships for young people to study planetary science,” Burkhart told Universe Today. “There were a lot of people there in the lobby buying posters autographed by Clyde Tombaugh and I wanted one very much.”
However, when Burkhart went to purchase one, he discovered that in his haste to leave his home, he had forgotten his billfold.
“I waited until everything was over and thought that I would at least go over and say hi to Clyde and tell him how much I thought of his hard work and to shake his hand, at least,” Burkhart said, and Tombaugh was more than happy to chat with an fellow astronomy enthusiast.
“While I was chatting with Clyde, I told him that I wish I brought money to buy one of the posters. He looked at me and smiled and said, ‘Well, that’s alright.’” And I said no, I really would have bought one if I had not ran out of the house and forgot my billfold. He was holding his notes and I asked him, what are you going to do with those notes, throw them away?”
Burkhart said Tombaugh smiled and replied that he couldn’t give away his notes, as he had more talks to give, but said he could mail them to Burkhart after his tour was over.
Burkhart offered to send Tombaugh a check later, or at least pay for postage, but Tombaugh looked at him and said, “No, that’s OK, I see you are really into astronomy and it would be my pleasure to give it you.”
Grateful, Burkhart asked if Tombaugh could autograph it, not for Burkhart but for his son Jason. Tombaugh took Burkhart’s address, and true to his word, about a month later Burkhart received Tombaugh’s personal version of the Commandments, with corrections made in pen, (the corrections were made by Tombaugh’s wife, Patricia, Burkhart said) along with his autograph. “I have them in safekeeping to leave to my son to have and hopefully give them to his kids,” Burkhart said.
Here are the the Ten Special Commandments for a Would-Be Planet Hunter, according to Clyde Tombaugh
1. Behold the heavens and the great vastness thereof, for a planet could be anywhere therein.
2. Thou shalt dedicate thy whole being to the search project with infinite patience and perseverance.
3. Though shalt set no other work before thee for the search shall keep thee busy enough.
4. Though shalt take the plates at opposition time lest thou be deceived by asteroids near their stationary positions.
5. Though shalt duplicate the plate of a pair at the same hour angle lest refraction distortions overtake thee.
6. Thou shalt give adequate overlap of adjacent plate regions lest the planet play hide and seek with thee.
7. Thou must not become ill in the dark of the moon lest thou fall behind the opposition point.
8. Thou shalt have no dates except at full moon when long exposure plates cannot be taken at the telescope.
9. Many false planets shall appear before thee, hundreds of them, and thou shalt check every one with a third plate.
10. Thou shalt not engage in any dissipation, that thy years may be many for thou shalt need them to finish the job!
Clyde W. Tombaugh
14 March 1989
Burkhart shared the scan of Tombaugh’s notes on his Facebook page.
h/t to Charles Bell.
Now is the Time for Observing Saturn in the Night Sky

[/caption]
April is Global Astronomy Month, and as a highlight, the “Lord of the Rings” is back prominently in the night sky! This past weekend, Saturn reached opposition, when it is closest to the Earth in its orbit. Opposition means Saturn is directly opposite the Sun in the sky, so it rises in the east at sunset and sets in the west at sunrise – meaning it will be in the sky all night long! Saturn is also the only planet visible before midnight in April. But the beautiful ringed planet will be looking even brighter that it has been for the past few months.
Special thanks to Efrain Morales Rivera for sending us this montage of Saturn images, showing a current view of Saturn in the middle and the ringed planet at oppositions from the last six years.
Around opposition there is a phenomenon known as the Seeliger Effect, which might actually be a couple of different effects combining to enhance the brightness of Saturn: Since the Earth is smack dab in the middle of the Sun and Saturn, sunlight is coming from directly behind us and directly at Saturn. And there’s also the phenomenon called coherent backscattering, which is where if you shine a beam of light at something that is made from a lot of separate particles (like Saturn’s rings) the light is reflected back with greater intensity from the direction directly opposite the beam. The middle image of Saturn in the montage above reveals brighter rings from the Seeliger Effect, as well as highlighting the ‘Serpent storm’ in the northern hemisphere.
You can read more about the Seeliger Effect on AstroBob’s website.
This is also a great time to try and see some of Saturn’s moons, too.
Astronomers Without Borders are sponsoring a special Saturn Watch event April 11-16. See their website for more information about “Saturn Watch” and activity suggestions.
Here are more Saturn resources.
There are also several other fun observing events for Global Astronomy Month:
On April 9 the Global Star Party will unfold as darkness sweeps around the Earth. This is the night to set up your telescopes and share the wonders of the sky with others.
From April 10 to 16 it’s Lunar Week, to turn your gaze turns toward Earth’s natural satellite, and take a close-up look the Moon’s craters and “seas”.
April 12th is Yuri’s Night — and a very special one too, as it is the 50th anniversary of the first human in space.
April 17 is SunDay, highlighting our very own star.
The month closes with the Lyrid Meteor shower. On April 21/22 get comfortable in something warm and spend
the night scanning the sky for meteors caused by debris left behind by Comet Thatcher.
See more on the Global Astronomy Month website.
You can hear a podcast about Dark Skies Month on the April 5th 365 Days of Astronomy podcast.
Kickstarting the Joy of Astronomy
[/caption]
You’re probably familiar with Sidewalk Astronomy – where amateur astronomers set up telescopes on street corners or other public places to share free views through a telescope with those who might not otherwise have the chance. These are great opportunities for public education about astronomy and the Universe in which we live. We just got a note from a long-time sidewalk astronomer, Jay Horowitz, who has a plan to set up telescopes on A LOT of street corners. He wants to share telescope views of the skies with people all over the US. But he needs a little help to make his plan come to fruition.
“ I want to give thousands of people the opportunity to see firsthand the universe in which we live, turning young minds on to the power of science and sparking curiosity and awe in adults,” Jay wrote us in an email. And so, he has set up a Kickstarter page – and you might be familiar with this new “crowd sourcing” way to fund creative projects.
Kickstarter projects are efforts by people to do something they love, something fun, or at least something of worthwhile and of note. But they might not have the funds to do it. Kickstarter is an all-or-nothing funding method where projects must be fully-funded or no money changes hands.
Check out Jay’s Astronomy On The Road Kickstarter project, where his goal is to take science around the country in 2011 and 2012. He’s looking to raise money for a Schmidt-Cassegrain telescope, a solar telescope, and fuel for transporting the telescopes between locations. He and his group will provide free views of the skies in schools, libraries, and on city streets all over the United States.
“The telescopes will always be used for public education and will never be for private use, even down the road,” Jay said.
There are also some notable prizes for those who donate, including lunch with some of the big names in astronomy.
Jay’s Sidewalk Astronomy resume is impressive: he founded a successful sidewalk astronomy group in New York City and also volunteered as a telescope operator and educator at an observatory, and taught astronomy in the Dominican Republic.
Consider donating to this great project. You can also follow the project on Twitter.