In recent years, the number of confirmed extra-solar planets has risen exponentially. As of the penning of the article, a total of 3,777 exoplanets have been confirmed in 2,817 star systems, with an additional 2,737 candidates awaiting confirmation. What’s more, the number of terrestrial (i.e. rocky) planets has increased steadily, increasing the likelihood that astronomers will find evidence of life beyond our Solar System.
Unfortunately, the technology does not yet exist to explore these planets directly. As a result, scientists are forced to look for what are known as “biosignatures”, a chemical or element that is associated with the existence of past or present life. According to a new study by an international team of researchers, one way to look for these signatures would be to examine material ejected from the surface of exoplanets during an impact event.
The study – titled “Searching for biosignatures in exoplanetary impact ejecta“, was published in the scientific journal Astrobiology and recently appeared online. It was led by Gianni Cataldi, a researcher from Stockholm University’s Astrobiology Center. He was joined by scientists from the LESIA-Observatoire de Paris, the Southwest Research Institute (SwRI), the Royal Institute of Technology (KTH), and the European Space Research and Technology Center (ESA/ESTEC).
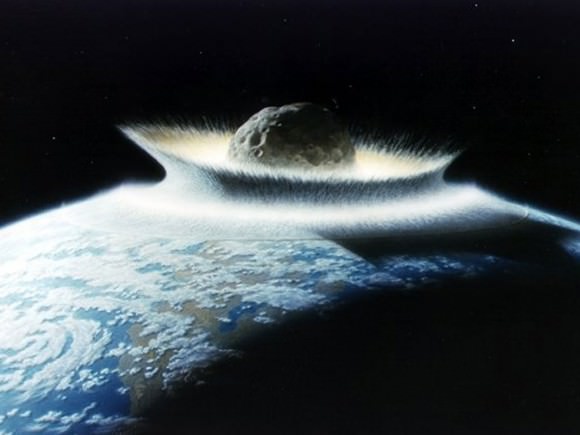
As they indicate in their study, most efforts to characterize exoplanet biospheres have focused on the planets’ atmospheres. This consists of looking for evidence of gases that are associated with life here on Earth – e.g. carbon dioxide, nitrogen, etc. – as well as water. As Cataldi told Universe Today via email:
“We know from Earth that life can have a strong impact on the composition of the atmosphere. For example, all the oxygen in our atmosphere is of biological origin. Also, oxygen and methane are strongly out of chemical equilibrium because of the presence of life. Currently, it is not yet possible to study the atmospheric composition of Earth-like exoplanets, however, such a measurement is expected to become possible in the foreseeable future. Thus, atmospheric biosignatures are the most promising way to search for extraterrestrial life.”
However, Cataldi and his colleagues considered the possibility of characterizing a planet’s habitability by looking for signs of impacts and examining the ejecta. One of the benefits of this approach is that ejecta escapes lower gravity bodies, such as rocky planets and moons, with the greatest ease. The atmospheres of these types of bodies are also very difficult to characterize, so this method would allow for characterizations that would not otherwise be possible.
And as Cataldi indicated, it would also be complimentary to the atmospheric approach in a number of ways:
“First, the smaller the exoplanet, the more difficult it is to study its atmosphere. On the contrary, smaller exoplanets produce larger amounts of escaping ejecta because their surface gravity is lower, making ejecta from smaller exoplanet easier to detect. Second, when thinking about biosignatures in impact ejecta, we think primarily of certain minerals. This is because life can influence the mineralogy of a planet either indirectly (e.g. by changing the composition of the atmosphere and thus allowing new minerals to form) or directly (by producing minerals, e.g. skeletons). Impact ejecta would thus allow us to study a different sort of biosignature, complementary to atmospheric signatures.”
Another benefit to this method is the fact that it takes advantage of existing studies that have examined the impacts of collisions between astronomical objects. For instance, multiple studies have been conducted that have attempted to place constraints on the giant impact that is believed to have formed the Earth-Moon system 4.5 billion years ago (aka. the Giant Impact Hypothesis).
While such giant collisions are thought to have been common during the final stage of terrestrial planet formation (lasting for approximately 100 million years), the team focused on impacts of asteroidal or cometary bodies, which are believed to occur over the entire lifetime of an exoplanetary system. Relying on these studies, Cataldi and his colleagues were able to create models for exoplanet ejecta.
As Cataldi explained, they used the results from the impact cratering literature to estimate the amount of ejecta created. To estimate the signal strength of circumstellar dust disks created by the ejecta, they used the results from debris disk (i.e. extrasolar analogues of the Solar System’s Main Asteroid Belt) literature. In the end, the results proved rather interesting:
“We found that an impact of a 20 km diameter body produces enough dust to be detectable with current telescopes (for comparison, the size of the impactor that killed the dinosaurs 65 million years ago is though to be around 10 km). However, studying the composition of the ejected dust (e.g. search for biosignatures) is not in the reach of current telescopes. In other words, with current telescopes, we could confirm the presence of ejected dust, but not study its composition.”
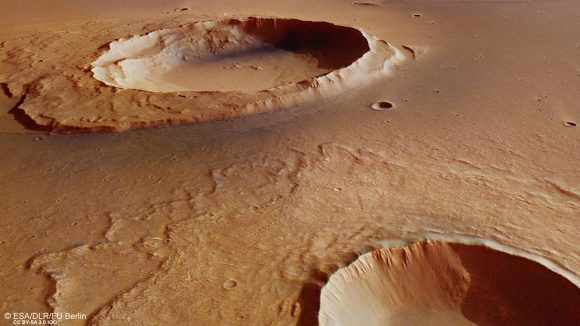
In short, studying material ejected from exoplanets is within our reach and the ability to study its composition someday will allow astronomers to be able to characterize the geology of an exoplanet – and thus place more accurate constraints on its potential habitability. At present, astronomers are forced to make educated guesses about a planet’s composition based on its apparent size and mass.
Unfortunately, a more detailed study that could determine the presence of biosignatures in ejecta is not currently possible, and will be very difficult for even next-generation telescopes like the James Webb Space Telescope (JWSB) or Darwin. In the meantime, the study of ejecta from exoplanets presents some very interesting possibilities when it comes to exoplanet studies and characterization. As Cataldi indicated:
“By studying the ejecta from an impact event, we could learn something about the geology and habitability of the exoplanet and potentially detect a biosphere. The method is the only way I know to access the subsurface of an exoplanet. In this sense, the impact can be seen as a drilling experiment provided by nature. Our study shows that dust produced in an impact event is in principle detectable, and future telescopes might be able to constrain the composition of the dust, and therefore the composition of the planet.”
In the coming decades, astronomers will be studying extra-solar planets with instruments of increasing sensitivity and power in the hopes of finding indications of life. Given time, searching for biosignatures in the debris around exoplanets created by asteroid impacts could be done in tandem with searchers for atmospheric biosignatures.
With these two methods combined, scientists will be able to say with greater certainty that distant planets are not only capable of supporting life, but are actively doing so!
Further Reading: Astrobiology, arXiv