We all fear black holes, but how many of them are there out there, really? Between the stellar mass black holes and the supermassive ones, just how much of our Universe is black holes?
There are two kinds of black holes in the Universe that we know of: There’s stellar mass black holes, formed from massive stars, and a supermassive black holes which lives at the hearts of galaxies.
About 1 in a 1000 stars have enough mass to become a black hole when they die. Our Milky Way has 100 billion stars, this means it could have up to 100 million stellar mass black holes. As there are hundreds of billions of galaxies in the observable Universe, there are lots, lots more out there. In fact, the math suggests there’s a new black hole forming every second or so. So just to recap, the entire Universe is about 1/1000th “regular flavor” stellar mass black holes.
Supermassive black holes are a slightly different story. Our central galactic black hole is about 26,000 light years away from us. Formally, it’s called Sagittarius A-star, but for our purposes I’m going to call it Kevin. Just so you know they don’t throw that term “supermassive” around for no reason, Kevin contains 4.1 million times the mass of the Sun.
Kevin is gigantic and horrible. We can only imagine what it’s like to be in the region of space near Kevin. What percentage of the galaxy do you think Kevin makes up, mass wise?
Kevin, whilst absolutely super-massive, is a tiny, tiny 1/10,000 of a percent of the Milky Way galaxy’s mass. So, to be precise, if we add Kevin’s mass to the mass of all the stellar mass black holes aka. “mini-Kevins”, we get a very minor 11/10000s of a %.
As it turns out this ratio holds up on a Universal scale and is approximately the same for all the mass in the Universe. So, 11 ten thousandths of a percent is the answer to the question. As far as we know.
Unless… dark matter is black holes. Dark matter accounts for more than ¾ of the mass of the Universe. It doesn’t absorb light or interact with matter in any way. We’re only aware of its presence through its gravitational influence.
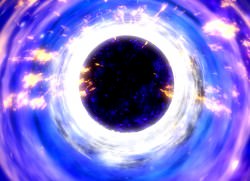
As it turns out, Astronomers think that one explanation for dark matter might be primordial black holes. These microscopic black holes would have the mass of an asteroid or more and could only form in the high pressure, high temperature conditions after the Big Bang.
Experiments to search for primordial black holes have yet to turn up any evidence, and most scientists don’t think they’re a viable explanation. But if they were, then the Universe is almost entirely composed of the physics inspired nightmare that are black holes.
If it’s not the case now, in the far future, everything could be. Given enough time, all those stellar black holes and supermassive Kevins will scoop up all the available material in the Universe.
In 10 quintillion years everything in the Universe will have either fallen into a black hole, or been flung out on an escape trajectory. And then those black holes will slowly evaporate over time, as predicted by Stephen Hawking.
In 10^66 years the smallest stellar black holes will have evaporated. The most massive supermassive black holes could take 10^100 years. And then, there won’t be any black holes at all.
What do you think? Is it mostly black holes or almost no black holes? Tell us what you suspect in the comments below.