For centuries, astronomers have been looking beyond our Solar System to learn more about the Milky Way Galaxy. And yet, there are still many things about it that elude us, such as knowing its precise mass. Determining this is important to understanding the history of galaxy formation and the evolution of our Universe. As such, astronomers have attempted various techniques for measuring the true mass of the Milky Way.
So far, none of these methods have been particularly successful. However, a new study by a team of researchers from the Harvard-Smithsonian Center for Astrophysics proposed a new and interesting way to determine how much mass is in the Milky Way. By using hypervelocity stars (HVSs) that have been ejected from the center of the galaxy as a reference point, they claim that we can constrain the mass of our galaxy.
Their study, titled “Constraining Milky Way Mass with Hypervelocity Stars“, was recently published in the journal Astronomy and Astrophysics. The study was produced by Dr. Giacomo Fragione, an astrophysicist at the University of Rome, and Professor Abraham Loeb – the Frank B. Baird, Jr. Professor of Science, the Chair of the Astronomy Department, and the Director of the Institute for Theory and Computation at Harvard University.
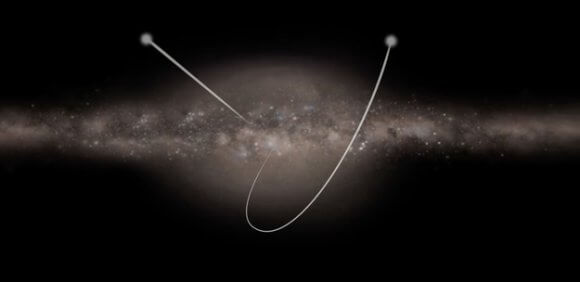
To be clear, determining the mass of the Milky Way Galaxy is no simple task. On the one hand, observations are difficult because the Solar System lies deep within the disk of the galaxy itself. But at the same time, there’s also the mass of our galaxy’s dark matter halo, which is difficult to measure since it is not “luminous”, and therefore invisible to conventional methods of detection.
Current estimates of the galaxy’s total mass are based on the motions of tidal streamers of gas and globular clusters, which are both influenced by the gravitational mass of the galaxy. But so far, these measurements have produced mass estimates that range from one to several trillion solar-masses. As Professor Loeb explained to Universe Today via email, precisely measuring the mass of the Milky Way is of great importance to astronomers:
“The Milky Way provides a laboratory for testing the standard cosmological model. This model predicts that the number of satellite galaxies of the Milky Way depends sensitively on its mass. When comparing the predictions to the census of known satellite galaxies, it is essential to know the Milky Way mass. Moreover, the total mass calibrates the amount of invisible (dark) matter and sets the depth of the gravitational potential well and implies how fast should stars move for them to escape to intergalactic space.”
For the sake of their study, Prof. Loeb and Dr. Fragione therefore chose to take a novel approach, which involved modeling the motions of HVSs to determine the mass of our galaxy. More than 20 HVSs have been discovered within our galaxy so far, which travel at speeds of up to 700 km/s (435 mi/s) and are located at distances of about 100 to 50,000 light-years from the galactic center.
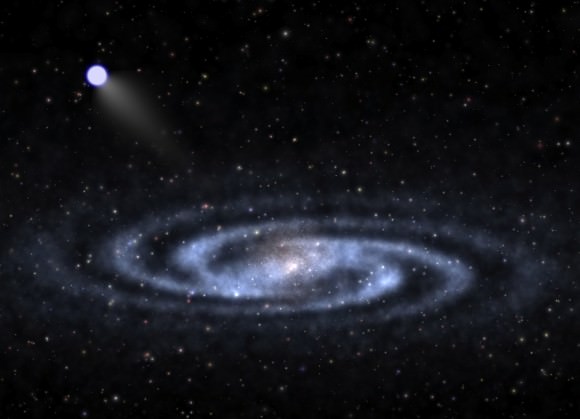
These stars are thought to have been ejected from the center of our galaxy thanks to the interactions of binary stars with the supermassive black hole (SMBH) at the center of our galaxy – aka. Sagittarius A*. While their exact cause is still the subject of debate, the orbits of HVSs can be calculated since they are completely determined by the gravitational field of the galaxy.
As they explain in their study, the researchers used the asymmetry in the radial velocity distribution of stars in the galactic halo to determine the galaxy’s gravitational potential. The velocity of these halo stars is dependent on the potential escape speed of HVSs, provided that the time it takes for the HVSs to complete a single orbit is shorter than the lifetime of the halo stars.
From this, they were able to discriminate between different models for the Milky Way and the gravitational force it exerts. By adopting the nominal travel time of these observed HVSs – which they calculated to about 330 million years, about the same as the average lifetime of halo stars – they were able to derive gravitational estimates for the Milky Way which allowed for estimates on its overall mass.
“By calibrating the minimum speed of unbound stars, we find that the Milky Way mass is in the range of 1.2-1.9 trillions solar masses,” said Loeb. While still subject to a range, this latest estimate is a significant improvement over previous estimates. What’s more, these estimates are consistent our current cosmological models that attempt to account for all visible matter in the Universe, as well as dark matter and dark energy – the Lambda-CDM model.
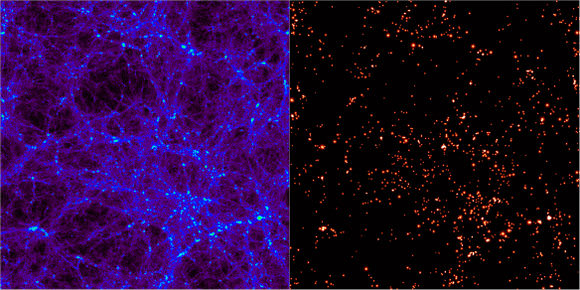
“The inferred Milky Way mass is in the range expected within the standard cosmological model,” said Leob, “where the amount of dark matter is about five times larger than that of ordinary (luminous) matter.”
Based on this breakdown, it can be said that normal matter in our galaxy – i.e. stars, planets, dust and gas – accounts for between 240 and 380 billion Solar Masses. So not only does this latest study provide more precise mass constraints for our galaxy, it could also help us to determine exactly how many star systems are out there – current estimates say that the Milky Way has between 200 to 400 billion stars and 100 billion planets.
Further Reading: Harvard Smithsonian CfA, Astronomy and Astrophysics