Margaret Geller is best known for her work on the large scale structure of the Universe, helping us understand the large clusters, super clusters and cosmic filaments that matter clumps into.
Continue reading “Astronomy Cast Ep. 359: Modern Women: Margaret Geller”
Orion Revisited: Astronomers Find New Star Cluster in Front of the Orion Nebula
The well-known star-forming region of the Orion Nebula. Credit: Canada-France-Hawaii Telescope / Coelum (J.-C. Cuillandre & G. Anselmi)
Precise distances are difficult to gauge in space, especially within the relatively local regions of the Galaxy. Stars which appear close together in the night sky may actually be separated by many hundreds or thousands of light-years, and since there’s only a limited amount of space here on Earth with which to determine distances using parallax, astronomers have to come up with other ways to figure out how far objects are, and what exactly is in front of or “behind” what.
Recently, astronomers using the 340-megapixel MegaCam on the Canada-France-Hawaii Telescope (CFHT) observed the star-forming region of the famous Orion nebula — located only about 1,500 light-years away — and determined that two massive groupings of the nebula’s stars are actually located in front of the cluster as completely separate structures… a finding that may ultimately force astronomers to rethink how the many benchmark stars located there had formed.
Although the Orion nebula is easily visible with the naked eye (as the hazy center “star” in Orion’s three-star sword, hanging perpendicular below his belt) its true nebulous nature wasn’t identified until 1610. As a vast and active star-forming region of bright dust and gas located a mere 1,500 light-years distant, the various stars within the Orion Nebula Cluster (ONC) has given astronomers invaluable benchmarks for research on many aspects of star formation.
[Read more: Astrophoto – Orion’s Bloody Massacre]
Now, CFHT observations of the Orion nebula conducted by Dr. Hervé Bouy of the European Space Astronomy Centre (ESAC) and Centre for Astrobiology (CSIC) and Dr. João Alves of the Institut für Astronomie (University of Vienna) have shown that a massive cluster of stars known as NGC 1980 is actually in front of the nebula, and is an older group of approximately 2,000 stars that is separate from the stars found within the ONC… as well as more massive than once thought.
“It is hard to see how these new observations fit into any existing theoretical model of cluster formation, and that is exciting because it suggests we might be missing something fundamental.”
– Dr. João Alves, Institut für Astronomie, University of Vienna
In addition their observations with CFHT — which were combined with previous observations with ESA’s Herschel and XMM-Newton and NASA’s Spitzer and WISE — have led to the discovery of another smaller cluster, L1641W.
According to the team’s paper, “We find that there is a rich stellar population in front of the Orion A cloud, from B-stars to M-stars, with a distinct 1) spatial distribution; 2) luminosity function; and 3) velocity dispersion from the reddened population inside the Orion A cloud. The spatial distribution of this population peaks strongly around NGC 1980 (iota Ori) and is, in all likelihood, the extended stellar content of this poorly studied cluster.”
The findings show that what has been known as Orion Nebula Cluster is actually a combination of older and newer groups of stars, possibly calling for a “revision of most of the observables in the benchmark ONC region (e.g., ages, age spread, cluster size, mass function, disk frequency, etc.)”
[Read more: Astronomers See Stars Changing Right Before Their Eyes in Orion Nebula]
“We must untangle these two mixed populations, star by star, if we are to understand the region, and star formation in clusters, and even the early stages of planet formation,” according to co-author Dr. Hervé Bouy.
The team’s article “Orion Revisited” was published in the November 2012 Astronomy & Astrophysics journal. Read the CFHT press release here.
The Canada-France-Hawaii Telescope’s Mauna Kea summit dome in September 2009. Credit: CFHT/Jean-Charles Cuillandre
Inset image: Orion nebula seen in optical – where the molecular cloud is invisible – and infrared, which shows the cloud. Any star detected in the optical in the line of sight over the region highlighted in the right panel must therefore be located in the foreground of the molecular cloud. Credit: J. Alves & H. Bouy.
Hot Gas Bridge Discovered Connecting Galaxy Clusters
An “bridge” of hot gas stretches between galaxy clusters Abell 401 and Abell 399
It may not be good practice to burn bridges but this is one super-heated bridge that astronomers were happy to find: an enormous swath of hot gas connecting two galaxy clusters 10 million light-years apart, and nearly a billion light-years away.
Using ESA’s Planck space telescope, astronomers have identified leftover light from the Big Bang interacting with a filament of hot gas stretching between Abell 401 and Abell 399, two galactic clusters each containing hundreds of individual galaxies.
Launched in May 2009, Planck is designed to study the Cosmic Microwave Background (CMB) — the leftover light from the Big Bang. When this radiation interacts with large-scale cosmic structures, like the hot gas bridging clusters of galaxies, its energy is modified in a specific way. This is referred to as the Sunyaev–Zel’dovich Effect (SZE), and Planck is specifically attuned to finding it.
This, however, is Planck’s first discovery of inter-cluster gas found using the SZ technique.
The temperature of the gas is estimated to be around 80 million degrees C, similar to the temperature of the gas found within the clusters themselves. It’s thought that the gas may be a combination of cosmic web filaments left over from the early Universe mixed with gas from the clusters.
The image above shows the clusters Abell 401 and Abell 399 as seen at optical wavelengths with ground-based telescopes overlaid with the SZE from Planck. The entire bridge spans a distance about the size of two full Moons in the sky.
Read more on ESA’s news page here.
Top image: Sunyaev–Zel’dovich effect: ESA Planck Collaboration; optical image: STScI Digitized Sky Survey. Inset image: Artist’s impression of Planck against the CMB. (ESA and the HFI Consortium, IRAS)
Do Stars Really Form in Clusters?
The long standing view on the formation of stars is that they form in clusters. This theory is supported by understanding of the formation process that requires large clouds of gas and dust to be able to condense. Small clouds with enough mass to only form one star just can’t meet the required conditions to condense. In a large cloud, where conditions are sufficient, once one star begins, the feedback effects from this star will trigger other star formation. Thus, if you get one, you’ll likely get lots.
But a new paper takes a critical look at whether or not all stars really form in clusters.
The main difficulty in answering this question boils down to a simple question: What does it mean to be “in” a cluster. Generally, members of a cluster are stars that are gravitationally bound. But as time passes, most clusters shed members as gravitational interactions, both internal and external, remove outer members. This blurs the boundary between being bound and unbound.
Similarly, some objects that can initially look very similar to clusters can actually be groups known as an association. As the name suggests, while these stars are in close proximity, they are not truly bond together. Instead, their relative velocities will cause the the group to disperse without the need for other effects.
As a result, astronomers have considered other requirements to truly be a member of a cluster. In particular for forming stars, there is an expectation that cluster stars should be able to interact with one-another during the formation process.
Its these considerations that this new team uses as a basis, led by Eli Bressert from the University of Exeter. Using observations from Spitzer, the team analyzed 12 nearby star forming regions. By conducting the survey with Spitzer, an infrared telescope, the team was able to pierce the dusty veil that typically hides such young stars.
By looking at the density of the young stellar objects (YSOs) in the plane of the sky, the team attempted to determine just what portion of stars could be considered true cluster members under various definitions. As might be expected, the answer was highly dependent on the definition used. If a loose and inclusive definition was taken, they determined that 90% of YSOs would be considered as part of the forming cluster. However, if the definition was drawn at the narrow end, the percentage dropped as low as 40%. Furthermore, if the additional criterion of needing to be in such proximity that their “formation/evolution (along with their circumstellar disks and/or planets) may be affected by the close proximity of their low-mass neighbours”, the percentage dropped to a scant 26%.
As with other definition boundaries, the quibbling may seem little more than a distraction. However, with such largely varying numbers attached to them, these triflings carry great significance since inconsistent definitions can greatly distort the understanding. This study highlights the need for clarity in definitions for which astronomers constantly struggle in a muddled universe full overlapping populations and shades of gray.
How to Crash Stars Together
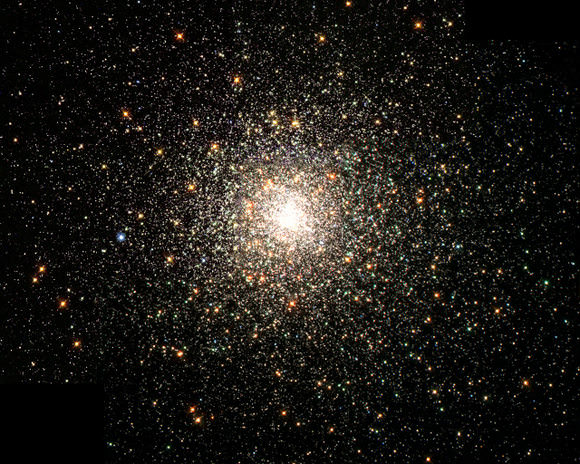
[/caption]
The math is simple: Star + Other star = Bigger star.
While conceptually this works well, it fails to take into account the extremely vast distances between stars. Even in clusters, where the density of stars is significantly higher than in the main disk, the number of stars per unit volume is so low that collisions are scarcely considered by astronomers. Of course, at some point the stellar density must reach a point at which the chance for a collision does become statistically significant. Where is that tipping point and are there any locations that might actually make the cut?
Early in the development of stellar formation models, the necessity of stellar collisions to produce massive stars was not well constrained. Early models of formation via accretion hinted that accretion may be insufficient, but as models became more complex and moved into three dimensional simulations, it became apparent that collisions simply weren’t needed to populate the upper mass regime. The notion fell out of favor.
However, there have been two recent papers that have explored the possibility that, while still certainly rare, there may be some environments in which collisions are likely to occur. The primary mechanism that assists in this is the notion that, as clusters sweep through the interstellar medium, they will inevitably pick up gas and dust, slowly increase in mass. This increase mass will cause the cluster to shrink, increasing the stellar density. The studies suggest that in order for the probability of collision to be statistically significant, a cluster would be required to reach a density of roughly 100 million stars per cubic parsec. (Keep in mind, a parsec is 3.26 light years and is roughly the distance between the sun, and our nearest neighboring star.)
Presently, such a high concentration has never been observed. While some of this is certainly due to the rarity of such densities, observational constraints likely play a crucial role in making such systems difficult to detect. If such high densities were to be achieved, it would require extraordinarily high spatial resolution to distinguish such systems. As such, numerical simulations of extremely dense systems will have to replace direct observations.
While the density necessary is straightforward, the more difficult topic is what sorts of clusters might be capable of meeting such criteria. To investigate this, the teams writing the recent papers conducted Monte Carlo simulations in which they could vary the numbers of stars. This type of simulation is essentially a model of a system that is allowed to play forward repeatedly with slightly different starting configurations (such as the initial positions of the stars) and by averaging the results of numerous simulations, an approximate understanding of the behavior of the system is reached. An initial investigation suggested that such densities could be reached in clusters with as little as a few thousand stars provided gas accumulation were sufficiently rapid (clusters tend to disperse slowly under tidal stripping which can counteract this effect on longer timescales). However, the model they used contained numerous simplifications since the investigation into the feasibility of such interactions was merely preliminary.
The more recent study, uploaded to arXiv yesterday, includes more realistic parameters and finds that the overall number of stars in the clusters would need to be closer to 30,000 before collisions became likely. This team also suggested that there were more conditions that would need to be satisfied including rates of gas expulsion (since not all gas would remain in the cluster as the first team had assumed for simplicity) and the degree of mass segregation (heavier stars sink to the center and lighter ones float to the outside and since heavier ones are larger, this actually decreases the number density while increasing the mass density). While many globular clusters can easily meet the requirement of number of stars, these other conditions would likely not be met. Furthermore, globular clusters spend little time in regions of the galaxy in which they would be likely to encounter sufficiently high densities of gas to allow for accumulation of sufficient mass on the necessary timescales.
But are there any clusters which might achieve sufficient density? The most dense galactic cluster known is the Arches cluster. Sadly, this cluster only reaches a modest ~535 stars per cubic parsec, still far too low to make a large number of collisions likely. However, one run of the simulation code with conditions similar to those in the Arches cluster did predict one collision in ~2 million years.
Overall, these studies seem to confirm that the role of collisions in forming massive stars is small. As pointed out previously, accretion methods seem to account for the broad range of stellar masses. Yet in many young clusters, still forming stars, rarely do astronomers find stars much in excess of ~50 solar masses. The second study this year suggests that this observation may yet leave room for collisions to play some unexpected role.
(NOTE: While it may be suggested that collisions could also be considered to take place as the orbit of binary stars decays due to tidal interactions, such processes are generally referred to as “mergers”. The term “collision” as used in the source materials and this article is used to denote the merging of two stars that are not gravitationally bound.)
Sources:
Stellar collisions in accreting protoclusters: a Monte Carlo dynamical study
Collisional formation of very massive stars in dense clusters
Dark Matter in Distant Galaxy Groups Mapped for the First Time
[/caption]
Galaxy density in the Cosmic Evolution Survey (COSMOS) field, with colors representing the redshift of the galaxies, ranging from redshift of 0.2 (blue) to 1 (red). Pink x-ray contours show the extended x-ray emission as observed by XMM-Newton.
Dark matter (actually cold, dark – non-baryonic – matter) can be detected only by its gravitational influence. In clusters and groups of galaxies, that influence shows up as weak gravitational lensing, which is difficult to nail down. One way to much more accurately estimate the degree of gravitational lensing – and so the distribution of dark matter – is to use the x-ray emission from the hot intra-cluster plasma to locate the center of mass.
And that’s just what a team of astronomers have recently done … and they have, for the first time, given us a handle on how dark matter has evolved over the last many billion years.
COSMOS is an astronomical survey designed to probe the formation and evolution of galaxies as a function of cosmic time (redshift) and large scale structure environment. The survey covers a 2 square degree equatorial field with imaging by most of the major space-based telescopes (including Hubble and XMM-Newton) and a number of ground-based telescopes.
Understanding the nature of dark matter is one of the key open questions in modern cosmology. In one of the approaches used to address this question astronomers use the relationship between mass and luminosity that has been found for clusters of galaxies which links their x-ray emissions, an indication of the mass of the ordinary (“baryonic”) matter alone (of course, baryonic matter includes electrons, which are leptons!), and their total masses (baryonic plus dark matter) as determined by gravitational lensing.
To date the relationship has only been established for nearby clusters. New work by an international collaboration, including the Max Planck Institute for Extraterrestrial Physics (MPE), the Laboratory of Astrophysics of Marseilles (LAM), and Lawrence Berkeley National Laboratory (Berkeley Lab), has made major progress in extending the relationship to more distant and smaller structures than was previously possible.
To establish the link between x-ray emission and underlying dark matter, the team used one of the largest samples of x-ray-selected groups and clusters of galaxies, produced by the ESA’s x-ray observatory, XMM-Newton.
Groups and clusters of galaxies can be effectively found using their extended x-ray emission on sub-arcminute scales. As a result of its large effective area, XMM-Newton is the only x-ray telescope that can detect the faint level of emission from distant groups and clusters of galaxies.
“The ability of XMM-Newton to provide large catalogues of galaxy groups in deep fields is astonishing,” said Alexis Finoguenov of the MPE and the University of Maryland, a co-author of the recent Astrophysical Journal (ApJ) paper which reported the team’s results.
Since x-rays are the best way to find and characterize clusters, most follow-up studies have until now been limited to relatively nearby groups and clusters of galaxies.
“Given the unprecedented catalogues provided by XMM-Newton, we have been able to extend measurements of mass to much smaller structures, which existed much earlier in the history of the Universe,” says Alexie Leauthaud of Berkeley Lab’s Physics Division, the first author of the ApJ study.
Gravitational lensing occurs because mass curves the space around it, bending the path of light: the more mass (and the closer it is to the center of mass), the more space bends, and the more the image of a distant object is displaced and distorted. Thus measuring distortion, or ‘shear’, is key to measuring the mass of the lensing object.
In the case of weak gravitational lensing (as used in this study) the shear is too subtle to be seen directly, but faint additional distortions in a collection of distant galaxies can be calculated statistically, and the average shear due to the lensing of some massive object in front of them can be computed. However, in order to calculate the lens’ mass from average shear, one needs to know its center.
“The problem with high-redshift clusters is that it is difficult to determine exactly which galaxy lies at the centre of the cluster,” says Leauthaud. “That’s where x-rays help. The x-ray luminosity from a galaxy cluster can be used to find its centre very accurately.”
Knowing the centers of mass from the analysis of x-ray emission, Leauthaud and colleagues could then use weak lensing to estimate the total mass of the distant groups and clusters with greater accuracy than ever before.
The final step was to determine the x-ray luminosity of each galaxy cluster and plot it against the mass determined from the weak lensing, with the resulting mass-luminosity relation for the new collection of groups and clusters extending previous studies to lower masses and higher redshifts. Within calculable uncertainty, the relation follows the same straight slope from nearby galaxy clusters to distant ones; a simple consistent scaling factor relates the total mass (baryonic plus dark) of a group or cluster to its x-ray brightness, the latter measuring the baryonic mass alone.
“By confirming the mass-luminosity relation and extending it to high redshifts, we have taken a small step in the right direction toward using weak lensing as a powerful tool to measure the evolution of structure,” says Jean-Paul Kneib a co-author of the ApJ paper from LAM and France’s National Center for Scientific Research (CNRS).
The origin of galaxies can be traced back to slight differences in the density of the hot, early Universe; traces of these differences can still be seen as minute temperature differences in the cosmic microwave background (CMB) – hot and cold spots.
“The variations we observe in the ancient microwave sky represent the imprints that developed over time into the cosmic dark-matter scaffolding for the galaxies we see today,” says George Smoot, director of the Berkeley Center for Cosmological Physics (BCCP), a professor of physics at the University of California at Berkeley, and a member of Berkeley Lab’s Physics Division. Smoot shared the 2006 Nobel Prize in Physics for measuring anisotropies in the CMB and is one of the authors of the ApJ paper. “It is very exciting that we can actually measure with gravitational lensing how the dark matter has collapsed and evolved since the beginning.”
One goal in studying the evolution of structure is to understand dark matter itself, and how it interacts with the ordinary matter we can see. Another goal is to learn more about dark energy, the mysterious phenomenon that is pushing matter apart and causing the Universe to expand at an accelerating rate. Many questions remain unanswered: Is dark energy constant, or is it dynamic? Or is it merely an illusion caused by a limitation in Einstein’s General Theory of Relativity?
The tools provided by the extended mass-luminosity relationship will do much to answer these questions about the opposing roles of gravity and dark energy in shaping the Universe, now and in the future.
Sources: ESA, and a paper published in the 20 January, 2010 issue of the Astrophysical Journal (arXiv:0910.5219 is the preprint)