If you want to hear what Mars has to say, you’re going to have to listen closely. Very closely.
Continue reading “According to Perseverance, Mars is Quiet… too Quiet”The Coast of Antarctica is Starting to Turn Green
The Antarctic Peninsula is the northernmost part of Antarctica, and has the mildest climate on the continent. In January, the warmest part of the year, the temperature averages 1 to 2 °C (34 to 36 °F). And it’s getting warmer.
Those warm temperatures allow snow algae to grow, and now scientists have used remote sensing to map those algae blooms.
Continue reading “The Coast of Antarctica is Starting to Turn Green”Today is the Highest Concentration of Atmospheric CO2 in Human History. 415 Parts Per Million. Last Time it Was This High, There Were Trees at the South Pole
Think about this for a minute: We humans and our emissions are helping turn back the climatological clock by 2 or 3 million years, possibly more. Not since that time, called the Pliocene Epoch, has the CO2 ppm risen above 400.
Way back then, the CO2 helped keep the Earth’s temperature 2 to 3 degrees C warmer than it is now. And the Earth was a much different place back then.
Continue reading “Today is the Highest Concentration of Atmospheric CO2 in Human History. 415 Parts Per Million. Last Time it Was This High, There Were Trees at the South Pole”It Looks Like Plate Tectonics Aren’t Required to Support Life

When looking for potentially-habitable extra-solar planets, scientists are somewhat restricted by the fact that we know of only one planet where life exists (i.e. Earth). For this reason, scientists look for planets that are terrestrial (i.e. rocky), orbit within their star’s habitable zones, and show signs of biosignatures such as atmospheric carbon dioxide – which is essential to life as we know it.
This gas, which is the largely result of volcanic activity here on Earth, increases surface heat through the greenhouse effect and cycles between the subsurface and the atmosphere through natural processes. For this reason, scientists have long believed that plate tectonics are essential to habitability. However, according to a new study by a team from Pennsylvania State University, this may not be the case.
The study, titled “Carbon Cycling and Habitability of Earth-Sized Stagnant Lid Planets“, was recently published in the scientific journal Astrobiology. The study was conducted by Bradford J. Foley and Andrew J. Smye, two assistant professors from the department of geosciences at Pennsylvania State University.
On Earth, volcanism is the result of plate tectonics and occurs where two plates collide. This causes subduction, where one plate is pushed beneath the other and deeper into the subsurface. This subduction changes the dense mantle into buoyant magma, which rises through the crust to the Earth’s surface and creates volcanoes. This process can also aid in carbon cycling by pushing carbon into the mantle.
Plate tectonics and volcanism are believe to have been central to the emergence of life here on Earth, as it ensured that our planet had sufficient heat to maintain liquid water on its surface. To test this theory, Professors Foley and Smye created models to determine how habitable an Earth-like planet would be without the presence of plate tectonics.
These models took into account the thermal evolution, crustal production and CO2 cycling to constrain the habitability of rocky, Earth-sized stagnant lid planets. These are planets where the crust consists of a single, giant spherical plate floating on mantle, rather than in separate pieces. Such planets are thought to be far more common than planets that experience plate tectonics, as no planets beyond Earth have been confirmed to have tectonic plates yet. As Prof. Foley explained in a Penn State News press release:
“Volcanism releases gases into the atmosphere, and then through weathering, carbon dioxide is pulled from the atmosphere and sequestered into surface rocks and sediment. Balancing those two processes keeps carbon dioxide at a certain level in the atmosphere, which is really important for whether the climate stays temperate and suitable for life.”
Essentially, their models took into account how much heat a stagnant lid planet’s climate could retain based on the amount of heat and heat-producing elements present when the planet formed (aka. its initial heat budget). On Earth, these elements include uranium which produces thorium and heat when it decays, which then decays to produce potassium and heat.
After running hundreds of simulations, which varied the planet’s size and chemical composition, they found that stagnant lid planets would be able to maintain warm enough temperatures that liquid water could exist on their surfaces for billions of years. In extreme cases, they could sustain life-supporting temperatures for up to 4 billion years, which is almost the age of the Earth.
As Smye indicated, this is due in part to the fact that plate tectonics are not always necessary for volcanic activity:
“You still have volcanism on stagnant lid planets, but it’s much shorter lived than on planets with plate tectonics because there isn’t as much cycling. Volcanoes result in a succession of lava flows, which are buried like layers of a cake over time. Rocks and sediment heat up more the deeper they are buried.”
The researchers also found that without plate tectonics, stagnant lid planets could still have enough heat and pressure to experience degassing, where carbon dioxide gas can escape from rocks and make its way to the surface. On Earth, Smye said, the same process occurs with water in subduction fault zones. This process increases based on the quantity of heat-producing elements present in the planet. As Foley explained:
“There’s a sweet spot range where a planet is releasing enough carbon dioxide to keep the planet from freezing over, but not so much that the weathering can’t pull carbon dioxide out of the atmosphere and keep the climate temperate.”
According to the researchers’ model, the presence and amount of heat-producing elements were far better indicators for a planet’s potential to sustain life. Based on their simulations, they found that the initial composition or size of a planet is very important for determining whether or not it will become habitable. Or as they put it, the potential habitability of a planet is determined at birth.
By demonstrating that stagnant lid planets could still support life, this study has the potential for greatly extending the range of what scientists consider to be potentially-habitable. When the James Webb Space Telescope (JWST) is deployed in 2021, examining the atmospheres of stagnant lid planets to determine the presence of biosignatures (like CO2) will be a major scientific objective.
Knowing that more of these worlds could sustain life is certainly good news for those who are hoping that we find evidence of extra-terrestrial life in our lifetimes.
Further Reading: PennState, Astrobiology
Weekly Space Hangout – February 10, 2017: Weekend Eclipse, Occultation and Comet 45P!
Host: Fraser Cain (@fcain)
Guests:
Paul M. Sutter (pmsutter.com / @PaulMattSutter)
Morgan Rehnberg (MorganRehnberg.com / @MorganRehnberg)
Dave Dickinson (www.astroguyz.com / @astroguyz)
Their stories this week:
Mars didn’t have enough CO2 to sustain liquid water
ISS is getting a commercial airlock
We use a tool called Trello to submit and vote on stories we would like to see covered each week, and then Fraser will be selecting the stories from there. Here is the link to the Trello WSH page (http://bit.ly/WSHVote), which you can see without logging in. If you’d like to vote, just create a login and help us decide what to cover!
If you would like to join the Weekly Space Hangout Crew, visit their site here and sign up. They’re a great team who can help you join our online discussions!
If you would like to sign up for the AstronomyCast Solar Eclipse Escape, where you can meet Fraser and Pamela, plus WSH Crew and other fans, visit our site linked above and sign up!
We record the Weekly Space Hangout every Friday at 12:00 pm Pacific / 3:00 pm Eastern. You can watch us live on Universe Today, or the Universe Today YouTube page<
How Do We Terraform Venus?
Continuing with our “Definitive Guide to Terraforming“, Universe Today is happy to present to our guide to terraforming Venus. It might be possible to do this someday, when our technology advances far enough. But the challenges are numerous and quite specific.
The planet Venus is often referred to as Earth’s “Sister Planet”, and rightly so. In addition to being almost the same size, Venus and Earth are similar in mass and have very similar compositions (both being terrestrial planets). As a neighboring planet to Earth, Venus also orbits the Sun within its “Goldilocks Zone” (aka. habitable zone). But of course, there are many key difference between the planets that make Venus uninhabitable.
For starters, it’s atmosphere over 90 times thicker than Earth’s, its average surface temperature is hot enough to melt lead, and the air is a toxic fume consisting of carbon dioxide and sulfuric acid. As such, if humans want to live there, some serious ecological engineering – aka. terraforming – is needed first. And given its similarities to Earth, many scientists think Venus would be a prime candidate for terraforming, even more so than Mars!
Over the past century, the concept of terraforming Venus has appeared multiple times, both in terms of science fiction and as the subject of scholarly study. Whereas treatments of the subject were largely fantastical in the early 20th century, a transition occurred with the beginning of the Space Age. As our knowledge of Venus improved, so too did the proposals for altering the landscape to be more suitable for human habitation.
Examples in Fiction:
Since the early 20th century, the idea of ecologically transforming Venus has been explored in fiction. The earliest known example is Olaf Stapleton’s Last And First Men (1930), two chapters of which are dedicated to describing how humanity’s descendants terraform Venus after Earth becomes uninhabitable; and in the process, commit genocide against the native aquatic life.
By the 1950s and 60s, owing to the beginning of the Space Age, terraforming began to appear in many works of science fiction. Poul Anderson also wrote extensively about terraforming in the 1950s. In his 1954 novel, The Big Rain, Venus is altered through planetary engineering techniques over a very long period of time. The book was so influential that the term term “Big Rain” has since come to be synonymous with the terraforming of Venus.
In 1991, author G. David Nordley suggested in his short story (“The Snows of Venus”) that Venus might be spun-up to a day-length of 30 Earth days by exporting its atmosphere of Venus via mass drivers. Author Kim Stanley Robinson became famous for his realistic depiction of terraforming in the Mars Trilogy – which included Red Mars, Green Mars and Blue Mars.
In 2012, he followed this series up with the release of 2312, a science fiction novel that dealt with the colonization of the entire Solar System – which includes Venus. The novel also explored the many ways in which Venus could be terraformed, ranging from global cooling to carbon sequestration, all of which were based on scholarly studies and proposals.
Proposed Methods:
The first proposed method of terraforming Venus was made in 1961 by Carl Sagan. In a paper titled “The Planet Venus“, he argued for the use of genetically engineered bacteria to transform the carbon in the atmosphere into organic molecules. However, this was rendered impractical due to the subsequent discovery of sulfuric acid in Venus’ clouds and the effects of solar wind.
In his 1991 study “Terraforming Venus Quickly“, British scientist Paul Birch proposed bombarding Venus’ atmosphere with hydrogen. The resulting reaction would produce graphite and water, the latter of which would fall to the surface and cover roughly 80% of the surface in oceans. Given the amount of hydrogen needed, it would have to harvested directly from one of the gas giant’s or their moon’s ice.
The proposal would also require iron aerosol to be added to the atmosphere, which could be derived from a number of sources (i.e. the Moon, asteroids, Mercury). The remaining atmosphere, estimated to be around 3 bars (three times that of Earth), would mainly be composed of nitrogen, some of which will dissolve into the new oceans, reducing atmospheric pressure further.
Another idea is to bombard Venus with refined magnesium and calcium, which would sequester carbon in the form of calcium and magnesium carbonates. In their 1996 paper, “The stability of climate on Venus“, Mark Bullock and David H. Grinspoon of the University of Colorado at Boulder indicated that Venus’ own deposits of calcium and magnesium oxides could be used for this process. Through mining, these minerals could be exposed to the surface, thus acting as carbon sinks.
However, Bullock and Grinspoon also claim this would have a limited cooling effect – to about 400 K (126.85 °C; 260.33 °F) and would only reduce the atmospheric pressure to an estimated 43 bars. Hence, additional supplies of calcium and magnesium would be needed to achieve the 8×1020 kg of calcium or 5×1020 kg of magnesium required, which would most likely have to be mined from asteroids.
The concept of solar shades has also been explored, which would involve using either a series of small spacecraft or a single large lens to divert sunlight from a planet’s surface, thus reducing global temperatures. For Venus, which absorbs twice as much sunlight as Earth, solar radiation is believed to have played a major role in the runaway greenhouse effect that has made it what it is today.
Such a shade could be space-based, located in the Sun–Venus L1 Lagrangian point, where it would prevent some sunlight from reaching Venus. In addition, this shade would also serve to block the solar wind, thus reducing the amount of radiation Venus’ surface is exposed to (another key issue when it comes to habitability). This cooling would result in the liquefaction or freezing of atmospheric CO², which would then be depsotied on the surface as dry ice (which could be shipped off-world or sequestered underground).
Alternately, solar reflectors could be placed in the atmosphere or on the surface. This could consist of large reflective balloons, sheets of carbon nanotubes or graphene, or low-albedo material. The former possibility offers two advantages: for one, atmospheric reflectors could be built in-situ, using locally-sourced carbon. Second, Venus’ atmosphere is dense enough that such structures could easily float atop the clouds.
NASA scientist Geoffrey A. Landis has also proposed that cities could be built above Venus’ clouds, which in turn could act as both a solar shield and as processing stations. These would provide initial living spaces for colonists, and would act as terraformers, gradually converting Venus’ atmosphere into something livable so the colonists could migrate to the surface.
Another suggestion has to do with Venus’ rotational speed. Venus rotates once every 243 days, which is by far the slowest rotation period of any of the major planets. As such, Venus’s experiences extremely long days and nights, which could prove difficult for most known Earth species of plants and animals to adapt to. The slow rotation also probably accounts for the lack of a significant magnetic field.
To address this, British Interplanetary Society member Paul Birch suggested creating a system of orbital solar mirrors near the L1 Lagrange point between Venus and the Sun. Combined with a soletta mirror in polar orbit, these would provide a 24-hour light cycle.
It has also been suggested that Venus’ rotational velocity could be spun-up by either striking the surface with impactors or conducting close fly-bys using bodies larger than 96.5 km (60 miles) in diameter. There is also the suggestion of using using mass drivers and dynamic compression members to generate the rotational force needed to speed Venus up to the point where it experienced a day-night cycle identical to Earth’s (see above).
Then there’s the possibility of removing some of Venus’ atmosphere, which could accomplished in a number of ways. For starters, impactors directed at the surface would blow some of the atmosphere off into space. Other methods include space elevators and mass accelerators (ideally placed on balloons or platforms above the clouds), which could gradually scoop gas from the atmosphere and eject it into space.
Potential Benefits:
One of the main reasons for colonizing Venus, and altering its climate for human settlement, is the prospect of creating a “backup location” for humanity. And given the range of choices – Mars, the Moon, and the Outer Solar System – Venus has several things going for it the others do not. All of these highlight why Venus is known as Earth’s “Sister Planet”.
For starters, Venus is a terrestrial planet that is similar in size, mass and composition to Earth. This is why Venus has similar gravity to Earth, which is about of what we experience 90% (or 0.904 g, to be exact. As a result, humans living on Venus would be at a far lower risk of developing health problems associated with time spent in weightlessness and microgravity environments – such as osteoporosis and muscle degeneration.
Venus’s relative proximity to Earth would also make transportation and communications easier than with most other locations in the solar system. With current propulsion systems, launch windows to Venus occur every 584 days, compared to the 780 days for Mars. Flight time is also somewhat shorter since Venus is the closest planet to Earth. At it’s closest approach, it is 40 million km distant, compared to 55 million km for Mars.
Another reason has to do with Venus’ runaway greenhouse effect, which is the reason for the planet’s extreme heat and atmospheric density. In testing out various ecological engineering techniques, our scientists would learn a great deal about their effectiveness. This information, in turn, will come in mighty handy in the ongoing fight against Climate Change here on Earth.
And in the coming decades, this fight is likely to become rather intense. As the NOAA reported in March of 2015, carbon dioxide levels in the atmosphere have now surpassed 400 ppm, a level not seen since the the Pliocene Era – when global temperatures and sea level were significantly higher. And as a series of scenarios computed by NASA show, this trend is likely to continue until 2100, with severe consequences.
In one scenario, carbon dioxide emissions will level off at about 550 ppm toward the end of the century, resulting in an average temperature increase of 2.5 °C (4.5 °F). In the second scenario, carbon dioxide emissions rise to about 800 ppm, resulting in an average increase of about 4.5 °C (8 °F). Whereas the increases predicted in the first scenario are sustainable, in the latter scenario, life will become untenable on many parts of the planet.
So in addition to creating a second home for humanity, terraforming Venus could also help to ensure that Earth remains a viable home for our species. And of course, the fact that Venus is a terrestrial planet means that it has abundant natural resources that could be harvested, helping humanity to achieve a “post-scarcity” economy.
Challenges:
Beyond the similarities Venus’ has with Earth (i.e. size, mass and composition), there are numerous differences that would make terraforming and colonizing it a major challenge. For one, reducing the heat and pressure of Venus’ atmosphere would require a tremendous amount of energy and resources. It would also require infrastructure that does not yet exist and would be very expensive to build.
For instance, it would require immense amounts of metal and advanced materials to build an orbital shade large enough to cool Venus’ atmosphere to the point that its greenhouse effect would be arrested. Such a structure, if positioned at L1, would also need to be four times the diameter of Venus itself. It would have to be assembled in space, which would require a massive fleet of robot assemblers.
In contrast, increasing the speed of Venus’s rotation would require tremendous energy, not to mention a significant number of impactors that would have to cone from the outer solar System – mainly from the Kuiper Belt. In all of these cases, a large fleet of spaceships would be needed to haul the necessary material, and they would need to be equipped with advanced drive systems that could make the trip in a reasonable amount of time.
Currently, no such drive systems exist, and conventional methods – ranging from ion engines to chemical propellants – are neither fast or economical enough. To illustrate, NASA’s New Horizons mission took more than 11 years to get make its historic rendezvous with Pluto in the Kuiper Belt, using conventional rockets and the gravity-assist method.
Meanwhile, the Dawn mission, which relied relied on ionic propulsion, took almost four years to reach Vesta in the Asteroid Belt. Neither method is practical for making repeated trips to the Kuiper Belt and hauling back icy comets and asteroids, and humanity has nowhere near the number of ships we would need to do this.
The same problem of resources holds true for the concept of placing solar reflectors above the clouds. The amount of material would have to be large and would have to remain in place long after the atmosphere had been modified, since Venus’s surface is currently completely enshrouded by clouds. Also, Venus already has highly reflective clouds, so any approach would have to significantly surpass its current albedo (0.65) to make a difference.
And when it comes to removing Venus’ atmosphere, things are equally challenging. In 1994, James B. Pollack and Carl Sagan conducted calculations that indicated that an impactor measuring 700 km in diameter striking Venus at high velocity would less than a thousandth of the total atmosphere. What’s more, there would be diminishing returns as the atmosphere’s density decreases, which means thousands of giant impactors would be needed.
In addition, most of the ejected atmosphere would go into solar orbit near Venus, and – without further intervention – could be captured by Venus’s gravitational field and become part of the atmosphere once again. Removing atmospheric gas using space elevators would be difficult because the planet’s geostationary orbit lies an impractical distance above the surface, where removing using mass accelerators would be time-consuming and very expensive.
Conclusion:
In sum, the potential benefits of terraforming Venus are clear. Humanity would have a second home, we would be able to add its resources to our own, and we would learn valuable techniques that could help prevent cataclysmic change here on Earth. However, getting to the point where those benefits could be realized is the hard part.
Like most proposed terraforming ventures, many obstacles need to be addressed beforehand. Foremost among these are transportation and logistics, mobilizing a massive fleet of robot workers and hauling craft to harness the necessary resources. After that, a multi-generational commitment would need to be made, providing financial resources to see the job through to completion. Not an easy task under the most ideal of conditions.
Suffice it to say, this is something that humanity cannot do in the short-run. However, looking to the future, the idea of Venus becoming our “Sister Planet” in every way imaginable – with oceans, arable land, wildlife and cities – certainly seems like a beautiful and feasible goal. The only question is, how long will we have to wait?
We have written many interesting articles about terraforming here at Universe Today. Here’s The Definitive Guide To Terraforming, Could We Terraform the Moon?, Should We Terraform Mars?, How Do We Terraform Mars? and Student Team Wants to Terraform Mars Using Cyanobacteria.
We’ve also got articles that explore the more radical side of terraforming, like Could We Terraform Jupiter?, Could We Terraform The Sun?, and Could We Terraform A Black Hole?
For more information, check out Terraforming Mars at NASA Quest! and NASA’s Journey to Mars.
And if you liked the video posted above, come check out our Patreon page and find out how you can get these videos early while helping us bring you more great content!
NASA’s Carbon Dioxide Greenhouse Gas Observatory Captures ‘First Light’ at Head of International ‘A-Train’ of Earth Science Satellites
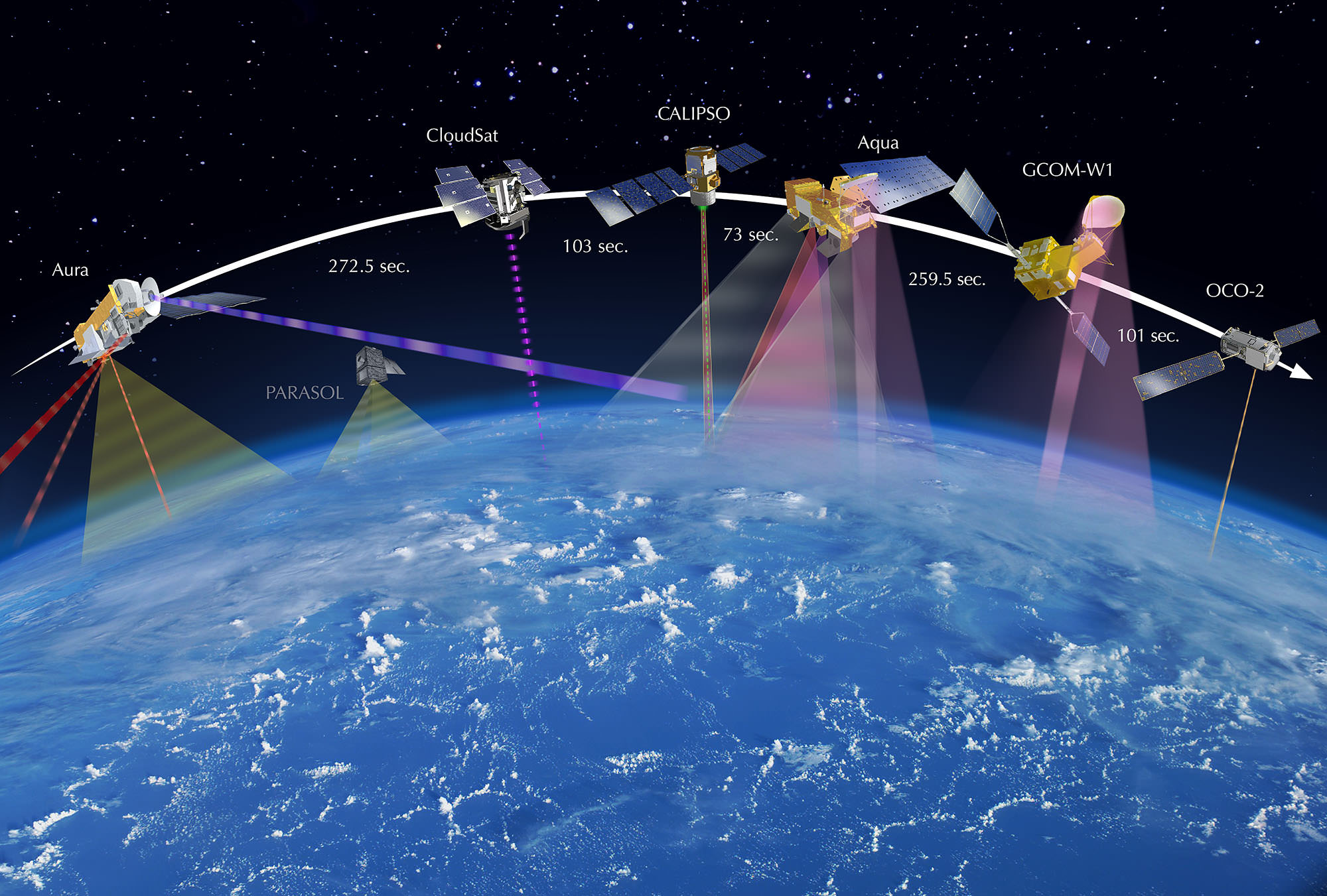
NASA’s first spacecraft dedicated to studying Earth’s atmospheric climate changing carbon dioxide (CO2) levels and its carbon cycle has reached its final observing orbit and taken its first science measurements as the leader of the world’s first constellation of Earth science satellites known as the International “A-Train.”
The Orbiting Carbon Observatory-2 (OCO-2) is a research satellite tasked with collecting the first global measurements of atmospheric carbon dioxide (CO2) – the leading human-produced greenhouse gas and the principal human-produced driver of climate change.
The ‘first light’ measurements were conducted on Aug. 6 as the observatory flew over central Papua New Guinea and confirmed the health of the science instrument. See graphic below.
NASA/JPL-Caltech/NASA GSFC
Before the measurements could begin, mission controllers had to cool the observatory’s three-spectrometer instrument to its operating temperatures.
“The spectrometer’s optical components must be cooled to near 21 degrees Fahrenheit (minus 6 degrees Celsius) to bring them into focus and limit the amount of heat they radiate. The instrument’s detectors must be even cooler, near minus 243 degrees Fahrenheit (minus 153 degrees Celsius), to maximize their sensitivity,” according to a NASA statement.
The team still has to complete a significant amount of calibration work before the observatory is declared fully operational.
OCO-2 was launched just over a month ago during a spectacular nighttime blastoff on July 2, 2014, from Vandenberg Air Force Base, California, atop a the venerable United Launch Alliance Delta II rocket.
OCO-2 arrived at its final 438-mile (705-kilometer) altitude, near-polar orbit on Aug. 3 at the head of the international A-Train following a series of propulsive burns during July. Engineers also performed a thorough checkout of all of OCO-2’s systems to ensure they were functioning properly.
“The initial data from OCO-2 appear exactly as expected — the spectral lines are well resolved, sharp and deep,” said OCO-2 chief architect and calibration lead Randy Pollock of JPL, in a statement.
“We still have a lot of work to do to go from having a working instrument to having a well-calibrated and scientifically useful instrument, but this was an important milestone on this journey.”
OCO-2 now leads the A-Train constellation, comprising five other international Earth orbiting monitoring satellites that constitute the world’s first formation-flying “super observatory” that collects an unprecedented quantity of nearly simultaneous climate and weather measurements.
Scientists will use the huge quantities of data to record the health of Earth’s atmosphere and surface environment as never before possible.
OCO-2 is followed in orbit by the Japanese GCOM-W1 satellite, and then by NASA’s Aqua, CALIPSO, CloudSat and Aura spacecraft, respectively. All six satellites fly over the same point on Earth within 16 minutes of each other. OCO-2 currently crosses the equator at 1:36 p.m. local time.
The 999 pound (454 kilogram) observatory is the size of a phone booth.
OCO-2 is equipped with a single science instrument consisting of three high-resolution, near-infrared spectrometers fed by a common telescope. It will collect global measurements of atmospheric CO2 to provide scientists with a better idea of how CO2 impacts climate change and is responsible for Earth’s warming.
During a minimum two-year mission the $467.7 million OCO-2 will take near global measurements to locate the sources and storage places, or ‘sinks’, for atmospheric carbon dioxide, which is a critical component of the planet’s carbon cycle.
OCO-2 was built by Orbital Sciences as a replacement for the original OCO which was destroyed during the failed launch of a Taurus XL rocket from Vandenberg back in February 2009 when the payload fairing failed to open properly and the spacecraft plunged into the ocean.
The OCO-2 mission will provide a global picture of the human and natural sources of carbon dioxide, as well as their “sinks,” the natural ocean and land processes by which carbon dioxide is pulled out of Earth’s atmosphere and stored, according to NASA.
Here’s a NASA description of how OCO-2 collects measurements.
As OCO-2 flies over Earth’s sunlit hemisphere, each spectrometer collects a “frame” three times each second, for a total of about 9,000 frames from each orbit. Each frame is divided into eight spectra, or chemical signatures, that record the amount of molecular oxygen or carbon dioxide over adjacent ground footprints. Each footprint is about 1.3 miles (2.25 kilometers) long and a few hundred yards (meters) wide. When displayed as an image, the eight spectra appear like bar codes — bright bands of light broken by sharp dark lines. The dark lines indicate absorption by molecular oxygen or carbon dioxide.
It will record around 100,000 precise individual CO2 measurements around the worlds entire sunlit hemisphere every day and help determine its source and fate in an effort to understand how human activities impact climate change and how we can mitigate its effects.
At the dawn of the Industrial Revolution, there were about 280 parts per million (ppm) of carbon dioxide in Earth’s atmosphere. As of today the CO2 level has risen to about 400 parts per million, which is the most in at least 800,000 years, says NASA.
OCO-2 is the second of NASA’s five new Earth science missions planned to launch in 2014 and is designed to operate for at least two years during its primary mission. It follows the successful blastoff of the joint NASA/JAXA Global Precipitation Measurement (GPM) Core Observatory satellite on Feb 27.
Stay tuned here for Ken’s continuing Earth and Planetary science and human spaceflight news.
A Dark and Dusty Avalanche on Mars
Mars may be geologically inactive but that doesn’t mean there’s nothing happening there — seasonal changes on the Red Planet can have some very dramatic effects on the landscape, as this recent image from the HiRISE camera shows!
When increasing light from the springtime Sun warms up the sides of sheer cliffs made from countless layers of water and carbon dioxide ice near Mars’ north pole, some of that CO2 ice sublimes, sending cascades of loose soil and dust down to the terraced base below. This uncovered material stains the frost-covered polar surface dark, outlining the paths of avalanches for HiRISE to easily spot from orbit. (See the original HiRISE image here.)
Circling Mars since March 2006, the HiRISE camera aboard NASA’s Mars Reconnaissance Orbiter has even captured some of these polar landslides in action.
The rust-colored avalanche shown above has fallen hundreds of meters from the middle of a layered ice deposit, spreading nearly a kilometer across the frozen ridges at the base of the cliff. The view was acquired on Sept. 13, 2013.
Check out a video explaining this view and the processes that created it below, narrated by Phil Plait (aka the Bad Astronomer).
Mars’ seasonal polar caps are composed primarily of carbon dioxide frost. This frost sublimates (changes from solid directly to gas) in the spring, boosting the pressure of Mars’ thin atmosphere. In the fall the carbon dioxide condenses, causing the polar caps to reach as far as ~55 degrees latitude by late winter. By learning about current processes on a local level we can learn more about how to interpret the geological record of climate changes on Mars. (Source)
Dry Ice Drives Dramatic Changes on Mars
Mars may not be tectonically active but that doesn’t mean there’s nothing happening on the Red Planet’s surface. This video from NASA’s Jet Propulsion Laboratory shows the dramatic seasonal changes that take place in Mars’ polar regions when the frozen carbon dioxide — called “dry ice” — coating the basalt sand dunes begins to thaw and cracks, releasing jets of sublimating CO2 gas that carry dark material upwards and outwards, staining the frozen surface of the dunes. Imagine what it would be like to be standing nearby when these jets erupt!
This process occurs around the upper latitudes of Mars every spring and is responsible for the dark (and sometimes light) mottled discolorations observed across sandy and dune-covered terrain.
Bright fans are created when surface conditions cause escaping CO2 gas to condense back onto the surface. (NASA/JPL/University of Arizona)
If a prevailing wind happens to be blowing when the gases are escaping the cracks in the ice, whatever material they are carrying will be spread by the wind across the dunes in long streaks and fans. Read more about this process here.
“It’s an amazingly dynamic process. We had this old paradigm that all the action on Mars was billions of years ago. Thanks to the ability to monitor changes with the Mars Reconnaissance Orbiter, one of the new paradigms is that Mars has many active processes today.”
– Candice Hansen, Planetary Science Institute
The images in the video were acquired by the HiRISE camera aboard the Mars Reconnaissance Orbiter, which has been orbiting and observing Mars in unprecedented detail for over six years. See more HiRISE images of the Martian surface here.
Video: NASA/JPL
Giant Spiders on Mars!
Eek, spiders! All right, so it’s not actually little green arachnids we’re talking about here, but they are definitely spidery features. Called araneiform terrain, these clusters of radially-branching cracks in Mars’ south polar surface are the result of the progressing spring season, when warmer temperatures thaw subsurface CO2 ice.
As dry ice below the surface warms it can sublimate rapidly and burst through the frozen ground above, creating long cracks. If the material below is dark it can be carried upwards by the escaping gas, staining the surface.
Each dark splotch is around 100 meters wide.
This image was acquired by the HiRISE camera aboard NASA’s Mars Reconnaissance Orbiter on September 26, from a distance of 262 km (163.8 miles). See the full-size scan here, and check out more recent HiRISE images in the November PDS release here.
Credit: NASA/JPL/University of Arizona