Sometimes when you stare at something long enough, you begin to see things. This is not the case with optical sensors and telescopes. Sure, there is noise from electronics, but it’s random and traceable. Stargazing with a telescope and camera is ideal for staring at the same patches of real estate for very long and repeated periods. This is the method used by the Dark Energy Survey (DES), and with less than one percent of the target area surveyed, astronomers are already discovering previously unknown objects in the outer Solar System.
The Dark Energy Survey is a five year collaborative effort that is observing Supernovae to better understand the structures and expansion of the universe. But in the meantime, transient objects much nearer to home are passing through the fields of view. Trans-Neptunian Objects (TNOs), small icy worlds beyond the planet Neptune, are being discovered. A new scientific paper, released as part of this year’s American Astronomical Society gathering in Seattle, Washington, discusses these newly discovered TNOs. The lead authors are two undergraduate students from Carleton College of Northfield, Minnesota, participating in a University of Michigan program.
The Palomar Sky Survey (POSS-1, POSS-2), the Sloan Digital Sky Survey, and every other sky survey have mapped not just the static, nearly unchanging night sky, but also transient events such as passing asteroids, comets, or novae events. The Dark Energy Survey is looking at the night sky for structures and expansion of the Universe. As part of the five year survey, DES is observing ten select 3 square degree fields for Type 1a supernovae on a weekly basis. As the survey proceeds, they are getting more than anticipated. The survey is revealing more trans-Neptunian objects. Once again, deep sky surveys are revealing more about our local environment – objects in the farther reaches of our Solar System.
DES is an optical imaging survey in search of Supernovae that can be used as weather vanes to measure the expansion of the universe. This expansion is dependent on the interaction of matter and the more elusive exotic materials of our Universe – Dark Energy and Dark Matter. The five year survey is necessary to achieve a level of temporal detail and a sufficient number of supernovae events from which to draw conclusions.
In the mean time, the young researchers of Carleton College – Ross Jennings and Zhilu Zhang – are discovering the transients inside our Solar System. Led by Professor David Gerdes of the University of Michigan, the researchers started with a list of nearly 100,000 observations of individual transients. Differencing software and trajectory analysis helped identify those objects that were trans-Neptunian rather than asteroids of the inner Solar System.
While asteroids residing in the inner solar system will pass quickly through such small fields, trans-Neptunian objects (TNOs) orbit the Sun much more slowly. For example, Pluto, at an approximate distance of 40 A.U. from the Sun, along with the object Eris, presently the largest of the TNOs, has an apparent motion of about 27 arc seconds per day – although for a half year, the Earth’s orbital motion slows and retrogrades Pluto’s apparent motion. The 27 arc seconds is approximately 1/60th the width of a full Moon. So, from one night to the next, TNOs can travel as much as 100 pixels across the field of view of the DES survey detectors since each pixel has a width of 0.27 arc seconds.
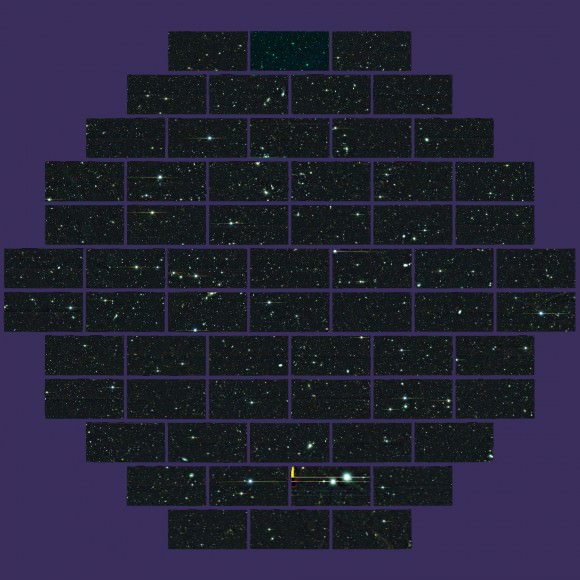
The scientific sensor array, DECam, is located at Cerro Tololo Inter-American Observatory (CTIO) in Chile utilizing the 4-meter (13 feet) diameter Victor M. Blanco Telescope. It is an array of 62 2048×4096 pixel back-illuminated CCDs totaling 520 megapixels, and altogether the camera weighs 20 tons.
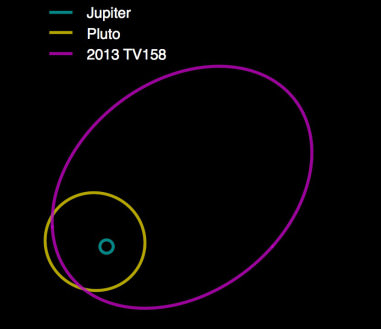
With a little over 2 years of observations, the young astronomers stated, “Our analysis revealed sixteen previously unknown outer solar system objects, including one Neptune Trojan, several objects in mean motion resonances with Neptune, and a distant scattered disk object whose 1200-year orbital period is among the 50 longest known.”

“So far we’ve examined less than one percent of the area that DES will eventually cover,” says Dr. Gerdes. “No other survey has searched for TNOs with this combination of area and depth. We could discover something really unusual.”
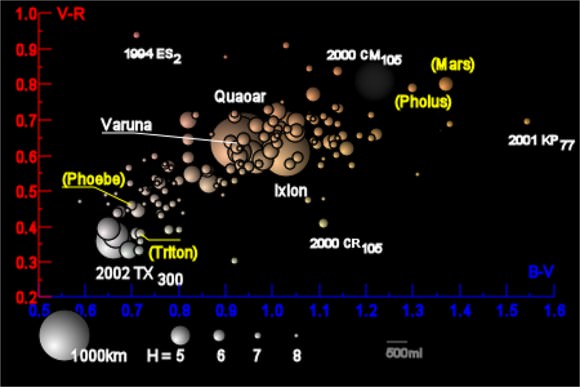
What does it all mean? It is further confirmation that the outer Solar System is chock-full of rocky-icy small bodies. There are other examples of recent discoveries, such as the search for a TNO for the New Horizons mission. As New Horizons has been approaching Pluto, the team turned to the Hubble space telescope to find a TNO to flyby after the dwarf planet. Hubble made short shrift of the work, finding three that the probe could reach. However, the demand for Hubble time does not allow long term searches for TNOs. A survey such as DES will serve to uncover many thousands of more objects in the outer Solar System. As Dr. Michael Brown of Caltech has stated, there is a fair likelihood that a Mars or Earth-sized object will be discovered beyond Neptune in the Oort Cloud.
References:
Observation of new trans-Neptunian Objects in the Dark Energy Survey Supernova Fields
Undergraduate Researchers Discover New Trans-Neptunian Objects
Dark Sky Detectives
For more details on the Dark Energy Survey: DES Website