The study of the Solar System’s many moons has revealed a wealth of information over the past few decades. These include the moons of Jupiter – 69 of which have been identified and named – Saturn (which has 62) and Uranus (27). In all three cases, the satellites that orbit these gas giants have prograde, low-inclination orbits. However, within the Neptunian system, astronomers noted that the situation was quite different.
Compared to the other gas giants, Neptune has far fewer satellites, and most of the system’s mass is concentrated within a single satellite that is believed to have been captured (i.e. Triton). According to a new study by a team from the Weizmann Institute of Science in Israel and the Southwest Research Institute (SwRI) in Boulder, Colorado, Neptune may have once had a more massive systems of satellites, which the arrival of Triton may have disrupted.
The study, titled “Triton’s Evolution with a Primordial Neptunian Satellite System“, recently appeared in The Astrophysical Journal. The research team consisted of Raluca Rufu, an astrophysicist and geophysicist from the Weizmann Institute, and Robin M. Canup – the Associate VP of the SwRI. Together, they considered models of a primordial Neptunian system, and how it may have changed thanks to the arrival of Triton.
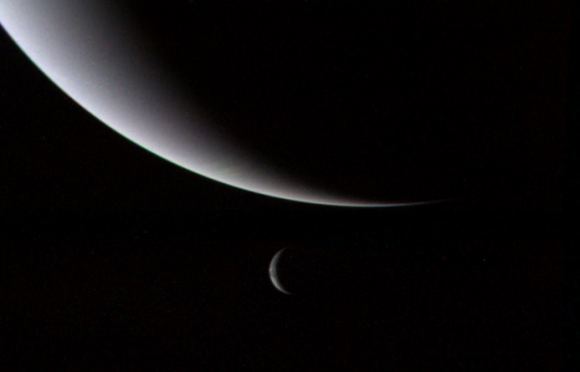
For many years, astronomers have been of the opinion that Triton was once a dwarf planet that was kicked out of the Kuiper Belt and captured by Neptune’s gravity. This is based on its retrograde and highly-inclined orbit (156.885° to Neptune’s equator), which contradicts current models of how gas giants and their satellites form. These models suggest that as giant planets accrete gas, their moons form from a surrounding debris disk.
Consistent with the other gas giants, the largest of these satellites would have prograde, regular orbits that are not particularly inclined relative to their planet’s equator (typically less than 1°). In this respect, Triton is believed to have once been part of a binary made up of two Trans-Neptunian Objects (TNOs). When they swung past Neptune, Triton would have been captured by its gravity and gradually fell into its current orbit.
As Dr. Rufu and Dr. Canup state in their study, the arrival of this massive satellite would have likely caused a lot of disruption in the Neptunian system and affected its evolution. This consisted of them exploring how interactions – like scattering or collisions – between Triton and Neptune’s prior satellites would have modified Triton’s orbit and mass, as well as the system at large. As they explain:
“We evaluate whether the collisions among the primordial satellites are disruptive enough to create a debris disk that would accelerate Triton’s circularization, or whether Triton would experience a disrupting impact first. We seek to find the mass of the primordial satellite system that would yield the current architecture of the Neptunian system.”
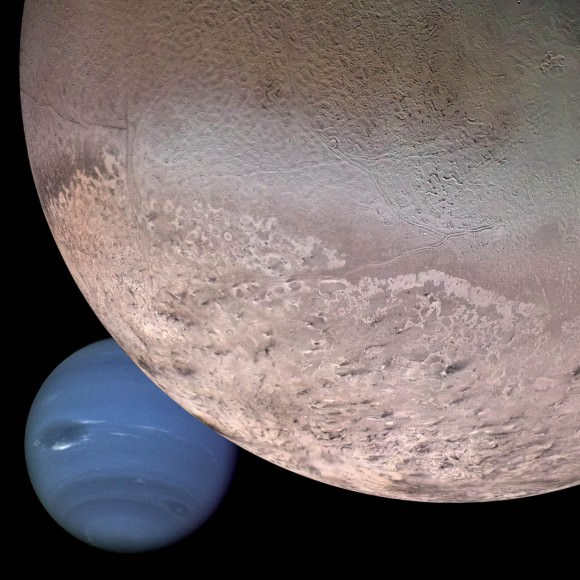
To test how the Neptunian system could have evolved, they considered different types of primordial satellite systems. This included one that was consistent with Uranus’ current system, made up of prograde satellites with a similar mass ration as Uranus’ largest moons – Ariel, Umbriel, Titania and Oberon – as well as one that was either more or less massive. They then conducted simulations to determine how Triton’s arrival would have altered these systems.
These simulations were based on disruption scaling laws which considered how non-hit-and-run impacts between Triton and other bodies would have led to a redistribution of matter in the system. What they found, after 200 simulations, was that a system that had a mass ratio that was similar to the current Uranian system (or smaller) would have been most likely to produce the current Neptunian system. As they state:
“We find that a prior satellite system with a mass ratio similar to the Uranian system or smaller has a substantial likelihood of reproducing the current Neptunian system, while a more massive system has a low probability of leading to the current configuration.”
They also found that the interaction of Triton with an earlier satellite system also offers a potential explanation for how its initial orbit could have been decreased fast enough to preserve the orbits of small irregular satellites. These Nereid-like bodies would have otherwise been kicked out of their orbits as tidal forces between Neptune and Triton caused Triton to assume its current orbit.
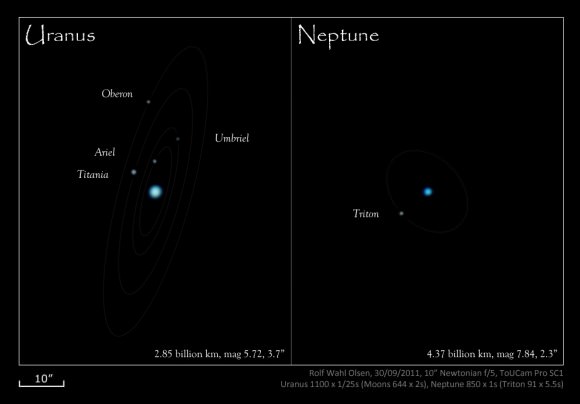
Ultimately, this study not only offers a possible explanation as to why Neptune’s system of satellites differs from those of other gas giants; it also indicates that Neptune’s proximity to the Kuiper Belt is what is responsible. At one time, Neptune may have had a system of moons that were very much like those of Jupiter, Saturn, and Uranus. But since it is well-situated to pick up dwarf planet-sized objects that were kicked out of the Kuiper Belt, this changed.
Looking to the future, Rufu and Canup indicate that additional studies are needed in order to shed light on Triton’s early evolution as a Neptunian satellite. Essentially, there are still unanswered questions concerning the effects the system of pre-existing satellites had on Triton, and how stable its irregular prograde satellites were.
These findings were also presented by Dr, Rufu and Dr. Canup during the 48th Lunar and Planetary Science Conference, which took place in The Woodlands, Texas, this past March.
Further Reading: The Astronomical Journal, USRA