Just a couple of weeks ago, astronomers from Caltech announced their third detection of gravitational waves from the Laser Interferometer Gravitational-Wave Observatory or LIGO.
As with the previous two detections, astronomers have determined that the waves were generated when two intermediate-mass black holes slammed into each other, sending out ripples of distorted spacetime.
One black hole had 31.2 times the mass of the Sun, while the other had 19.4 solar masses. The two spiraled inward towards each other, until they merged into a single black hole with 48.7 solar masses. And if you do the math, twice the mass of the Sun was converted into gravitational waves as the black holes merged.
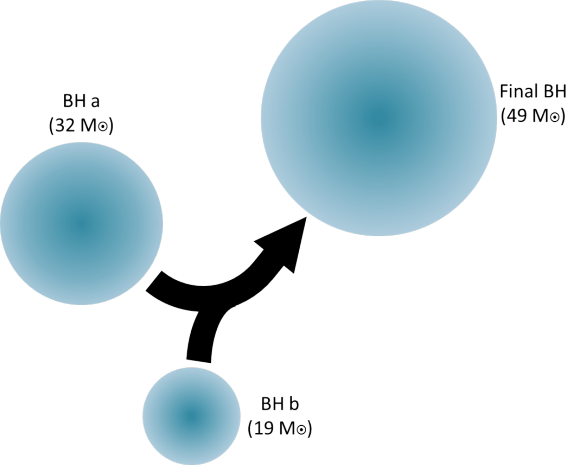
These gravitational waves traveled outward from the colossal collision at the speed of light, stretching and compressing spacetime like a tsunami wave crossing the ocean until they reached Earth, located about 2.9 billion light-years away.
The waves swept past each of the two LIGO facilities, located in different parts of the United States, stretching the length of carefully calibrated laser measurements. And from this, researchers were able to detect the direction, distance and strength of the original merger.
Seriously, if this isn’t one of the coolest things you’ve ever heard, I’m clearly easily impressed.
Now that the third detection has been made, I think it’s safe to say we’re entering a brand new field of gravitational astronomy. In the coming decades, astronomers will use gravitational waves to peer into regions they could never see before.
Being able to perceive gravitational waves is like getting a whole new sense. It’s like having eyes and then suddenly getting the ability to perceive sound.
This whole new science will take decades to unlock, and we’re just getting started.
As Einstein predicted, any mass moving through space generates ripples in spacetime. When you’re just walking along, you’re actually generating tiny ripples. If you can detect these ripples, you can work backwards to figure out what size of mass made the ripples, what direction it was moving, etc.
Even in places that you couldn’t see in any other way. Let me give you a couple of examples.
Black holes, obviously, are the low hanging fruit. When they’re not actively feeding, they’re completely invisible, only detectable by how they gravitational attract objects or bend light from objects passing behind them.
But seen in gravitational waves, they’re like ships moving across the ocean, leaving ripples of distorted spacetime behind them.
With our current capabilities through LIGO, astronomers can only detect the most massive objects moving at a significant portion of the speed of light. A regular black hole merger doesn’t do the trick – there’s not enough mass. Even a supermassive black hole merger isn’t detectable yet because these mergers seem to happen too slowly.
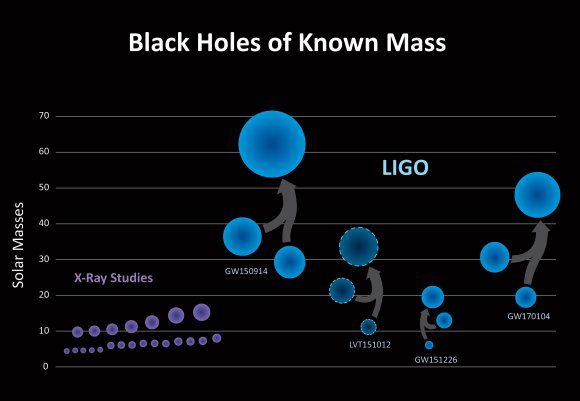
This is why all the detections so far have been intermediate-mass black holes with dozens of times the mass of our Sun. And we can only detect them at the moment that they’re merging together, when they’re generating the most intense gravitational waves.
If we can boost the sensitivity of our gravitational wave detectors, we should be able to spot mergers of less and more massive black holes.
But merging isn’t the only thing they do. Black holes are born when stars with many more times the mass of our Sun collapse in on themselves and explode as supernovae. Some stars, we’ve now learned just implode as black holes, never generating the supernovae, so this process happens entirely hidden from us.
Is there a singularity at the center of a black hole event horizon, or is there something there, some kind of object smaller than a neutron star, but bigger than an infinitely small point? As black holes merge together, we could see beyond the event horizon with gravitational waves, mapping out the invisible region within to get a sense of what’s going on down there.
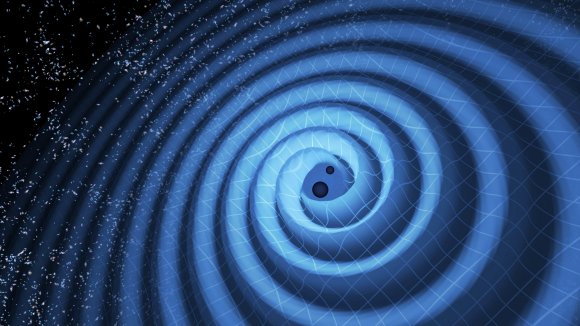
We want to know about even less massive objects like neutron stars, which can also form from a supernova explosion. These neutron stars can orbit one another and merge generating some of the most powerful explosions in the Universe: gamma ray bursts. But do neutron stars have surface features? Different densities? Could we detect a wobble in the gravitational waves in the last moments before a merger?
And not everything needs to merge. Sensitive gravitational wave detectors could sense binary objects with a large imbalance, like a black hole or neutron star orbiting around a main sequence star. We could detect future mergers by their gravitational waves.
Are gravitational waves a momentary distortion of spacetime, or do they leave some kind of permanent dent on the Universe that we could trace back? Will we see echoes of gravity from gravitational waves reflecting and refracting through the fabric of the cosmos?
Perhaps the greatest challenge will be using gravitational waves to see beyond the Cosmic Microwave Background Radiation. This region shows us the Universe 380,000 years after the Big Bang, when everything was cool enough for light to move freely through the Universe.
But there was mass there, before that moment. Moving, merging mass that would have generated gravitational waves. As we explained in a previous article, astronomers are working to find the imprint of these gravitational waves on the Cosmic Microwave Background, like an echo, or a shadow. Perhaps there’s a deeper Cosmic Gravitational Background Radiation out there, one which will let us see right to the beginning of time, just moments after the Big Bang.
And as always, there will be the surprises. The discoveries in this new field that nobody ever saw coming. The “that’s funny” moments that take researchers down into whole new fields of discovery, and new insights into how the Universe works.
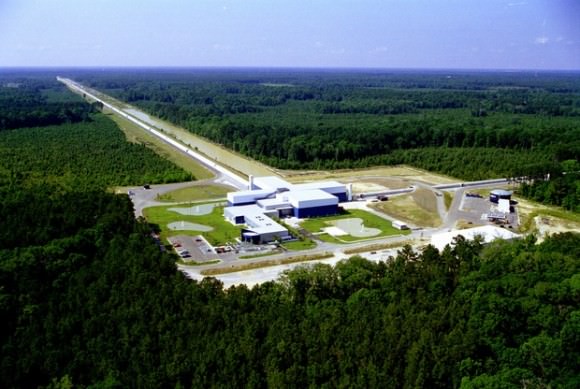
The LIGO project was begun back in 1994, and the first iteration operated from 2002 to 2012 without a single gravitational wave detection. It was clear that the facility wasn’t sensitive enough, so researchers went back and made massive improvements.
In 2008, they started improving the facility, and in 2015, Advanced LIGO came online with much more sensitivity. With the increased capabilities, Advanced LIGO made its first discovery in 2016, and now two more discoveries have been added.
LIGO can currently only detect the general hemisphere of the sky where a gravitational wave was emitted. And so, LIGO’s next improvement will be to add another facility in India, called INDIGO. In addition to improving the sensitivity of LIGO, this will give astronomers three observations of each event, to precisely detect the origin of the gravitational waves. Then visual astronomers could do follow up observations, to map the event to anything in other wavelengths.
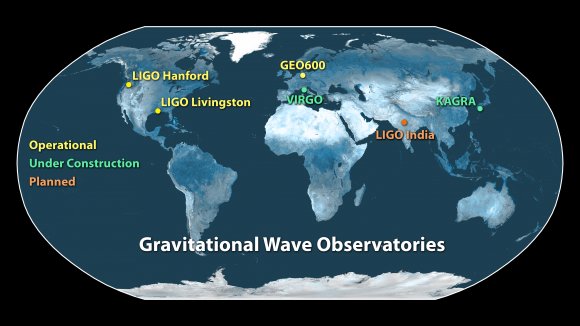
A European experiment known as Virgo has been operating for a few years as well, agreeing to collaborate with the LIGO team if any detections are made. So far, the Virgo experiment hasn’t found anything, but it’s being upgraded with 10 times the sensitivity, which should be fully operational by 2018.
A Japanese experiment called the Kamioka Gravitational Wave Detector, or KAGRA, will come online in 2018 as well, and be able to contribute to the observations. It should be capable of detecting binary neutron star mergers out to nearly a billion light-years away.
Just with visual astronomy, there are a set of next generation supergravitational wave telescopes in the works, which should come online in the next few decades.
The Europeans are building the Einstein Telescope, which will have detection arms 10 km long, compared to 4 km for LIGO. That’s like, 6 more km.
There’s the European Space Agency’s space-based Laser Interferometer Space Antenna, or LISA, which could launch in 2030. This will consist of a fleet of 3 spacecraft which will maintain a precise distance of 2.5 million km from each other. Compare that to the Earth-based detection distances, and you can see why the future of observations will come from space.
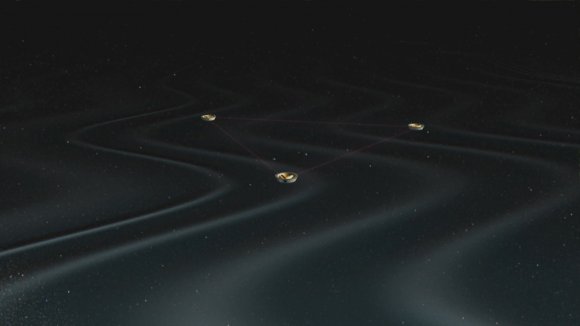
And that last idea, looking right back to the beginning of time could be a possibility with the Big Bang Observer mission, which will have a fleet of 12 spacecraft flying in formation. This is still all in the proposal stage, so no concrete date for if or when they’ll actually fly.
Gravitational wave astronomy is one of the most exciting fields of astronomy. This entirely new sense is pushing out our understanding of the cosmos in entirely new directions, allowing us to see regions we could never even imagine exploring before. I can’t wait to see what happens next.