In physics, there are two main ways to model the universe. The first is the classical way. Classical models such as Newton’s laws of motion and Einstein’s theory of relativity assume that the properties of an object such as its position and motion are absolute. There are practical limits to how accurately we can measure an object’s path through space and time, but that’s on us. Nature knows their motion with infinite precision. Quantum models such as atomic physics assume that objects are governed by interactions. These interactions are probabilistic and indefinite. Even if we constrain an interaction to limited outcomes, we can never know the motion of an object with infinite precision, because nature doesn’t allow it.
Continue reading “A new Approach Could Tease out the Connection Between Gravity and Quantum Mechanics”Magnetic Fields Help Shape the Formation of New Planets
In all of scientific modeling, the models attempting to replicate planetary and solar system formation are some of the most complicated. They are also notoriously difficult to develop. Normally they center around one of two formative ideas: planets are shaped primarily by gravity or planets are shaped primarily by magnetism. Now a new theoretical model has been developed by a team at the University of Zurich (UZH) that uses math from both methodologies to inform the most complete model yet of planetary formation.
Continue reading “Magnetic Fields Help Shape the Formation of New Planets”Health Issues From Spaceflight Might Originate in the Mitochondria
It’s not easy living and working in space for extended periods of time. As NASA’s Twins Study illustrated, microgravity takes a toll on human physiology, which is followed by a painful transition back to normal gravity (just ask Scott Kelly!) Aside from muscle and bone degeneration, there’s diminished organ function, effects on cardiovascular health, the central nervous system, and “subtle changes” on the genetic level.
Until now, the biggest unanswered question was what the underlying cause of these physical impacts was. But after reviewing all of the data accumulated from decades of research aboard the International Space Station (ISS) – which included the Twins Study and DNA samples taken from dozens of astronauts – an international team of researchers came to the conclusion that mitochondria might be the driving force for these changes.
Continue reading “Health Issues From Spaceflight Might Originate in the Mitochondria”Weekly Space Hangout: September 30, 2020, Dr. John Kiss Discusses the Growth of Plants in Space
This week, we welcome Dr. John Kiss from the University of North Carolina, Greensboro (UNCG). Tonight, Dr. Kiss will be discussing the sensory physiology of plants in space research, including the effect of Mars’ levels of gravity on plant development.
Continue reading “Weekly Space Hangout: September 30, 2020, Dr. John Kiss Discusses the Growth of Plants in Space”Gravity is tested down to a scale smaller than the thickness of a human hair
Gravity was the first force of nature to be realized, and in the centuries since we first cracked the code of that all-pervasive pulling power, scientists have continually come up with clever ways to test our understanding. And it’s no surprise why: the discovery of a new wrinkle in the gravitational force could open up vistas of new physics, and maybe even the nature of reality itself.
Continue reading “Gravity is tested down to a scale smaller than the thickness of a human hair”Astronomy Cast Ep. 503: Gravity Mapping
The Earth looks like a perfect sphere, but down here on the surface we see that there are mountains, rivers, oceans, glaciers, all kinds of features with different densities and shapes. Scientists can map this produce a highly detailed gravity map of our planet. And it turns out, this is very useful for other worlds too.
We usually record Astronomy Cast every Friday at 3:00 pm EST / 12:00 pm PST / 20:00 PM UTC. You can watch us live on AstronomyCast.com, or the AstronomyCast YouTube page.
Visit the Astronomy Cast Page to subscribe to the audio podcast!
If you would like to support Astronomy Cast, please visit our page at Patreon here – https://www.patreon.com/astronomycast. We greatly appreciate your support!
If you would like to join the Weekly Space Hangout Crew, visit their site here and sign up. They’re a great team who can help you join our online discussions!
Stars Orbiting Supermassive Black Hole Show Einstein was Right Again!
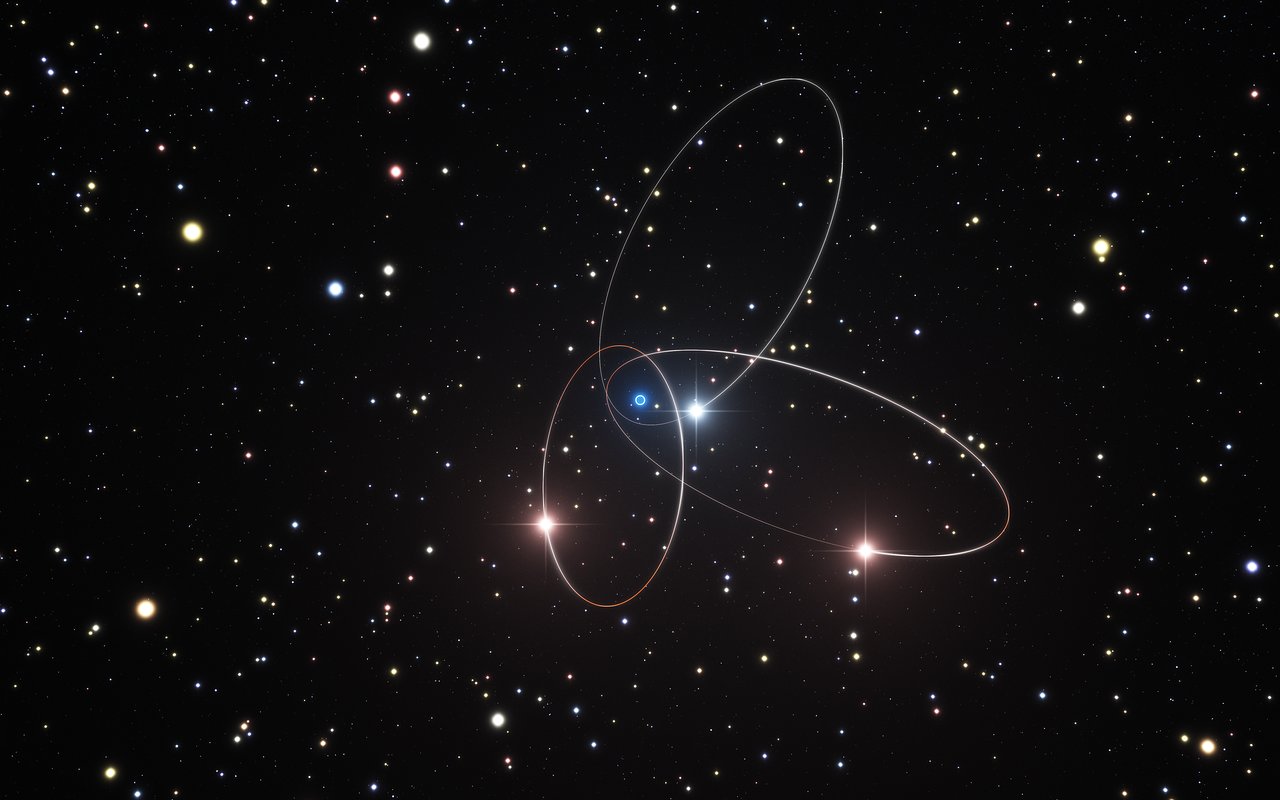
At the center of our galaxy, roughly 26,000 light years from Earth, lies the Supermassive Black Hole (SMBH) known as Sagittarius A*. Measuring 44 million km across, this object is roughly 4 million times as massive as our Sun and exerts a tremendous gravitational pull. Since astronomers cannot detect black holes directly, its existence has been determined largely from the effect it has on the small group of stars orbiting it.
In this respect, scientists have found that observing Sagittarius A* is an effective way of testing the physics of gravity. For instance, in the course of observing these stars, a team of German and Czech astronomers noted subtle effects caused by the black hole’s gravity. In so doing, they were able to yet again confirm some of the predictions made by Einstein’s famous Theory of General Relativity.
Their study, titled “Investigating the Relativistic Motion of the Stars Near the Supermassive Black Hole in the Galactic Center“, was recently published in the Astrophysical Journal. As is indicated in the course of it, the team applied new analysis techniques to existing observations that were made by European Southern Observatory’s (ESO) Very Large Telescope (VLT) and other telescopes over the course of the past 20 years.
From this, they measured the orbits of the stars that orbit Sagittarius A* to test predictions made by classical Newtonian physics (i.e. Universal Gravitation), as well as predictions based on general relativity. What they found was that one of the stars (S2) showed deviations in its orbit which were defied the former, but were consistent with the latter.
This star, which has 15 times the mass of our Sun, follows an elliptical orbit around the SMBH, completing a single orbit in about 15.6 years. At its closest, it gets to within 17 light hours of the black hole, which is the equivalent of 120 times the distance between the Sun and the Earth (120 AU). Essentially, the research team noted that S2 had the most elliptical orbit of any star orbiting the Supermassive Black Hole.
They also noted a slight change in its orbit – a few percent in the shape and about one-sixth of a degree in orientation. This could only be explained as being due to the relativistic effects caused by Sagittarius A* intense gravity, which cause a precession in its orbit. What this means is, the elliptical loop of S2’s orbit rotates around the SMBH over time, with its perihelion point aimed in different directions.
Interestingly enough, this is similar to the effect that was observed in Mercury’s orbit – aka. the “perihelion precession of Mercury” – during the late 19th century. This observation challenged classical Newtonian mechanics and led scientists to conclude that Newton’s theory of gravity was incomplete. It is also what prompted Einstein to develop his theory of General Relativity, which offered a satisfactory explanation for the issue.
Should the results of their study be confirmed, this will be the first time that the effects of general relativity have been precisely calculated using the stars that orbit a Supermassive Black Hole. Marzieh Parsa – a PhD student at the University of Cologne, Germany and lead author of the paper – was understandably excited with these results. As she stated in an ESO press statement:
“The Galactic Center really is the best laboratory to study the motion of stars in a relativistic environment. I was amazed how well we could apply the methods we developed with simulated stars to the high-precision data for the innermost high-velocity stars close to the supermassive black hole.“
This study was made possible thanks to the high-accuracy of the VLT’s instruments; in particular, the adaptive optics on the NACO camera and the SINFONI near-infrared spectrometer. These instruments were vital in tracking the star’s close approach and retreat from the black hole, which allowed for the team to precisely determine the shape of its orbit and thusly determine the relativistic effects on the star.
In addition to the more precise information about S2’s orbit, the team’s analysis also provided new and more accurate estimates of Sagittarius A* mass, as well as its distance from Earth. This could open up new avenues of research for this and other Supermassive Black Holes, as well as additional experiments that could help scientists to learn more about the physics of gravity.
The results also provided a preview of the measurements and tests that will be taking place next year. In 2018, the star S2 will be making a very close approach to Sagittarius A*. Scientists from around the world will be using this opportunity to test the GRAVITY instrument, a second-generation instrument that was recently installed on the Very Large Telescope Interferometer (VLTI).
Developed by an international consortium led by the Max Planck Institute for Extraterrestrial Physics, this instrument has been conducting observations of the Galactic Center since 2016. In 2018, it will be used to measure the orbit of S2 with even greater precision, which is expected to be most revealing. At this time, astrophysicists will be seeking to make additional measurements of the SMBH’s general relativistic effects.
Beyond that, they also hope to detect additional deviations in the star’s orbit that could hint at the existence of new physics! With the right tools trained on the right place, and at the right time, scientists just might find that even Einstein’s theories of gravity were not entirely complete. But in the meantime, it looks like the late and great theoretical physicist was right again!
And be sure to check out this video of the recent study, courtesy of the ESO:
Further Reading: ESO, Astrophysical Journal
Mouse Sperm Went to Space and Produced Healthy Mice
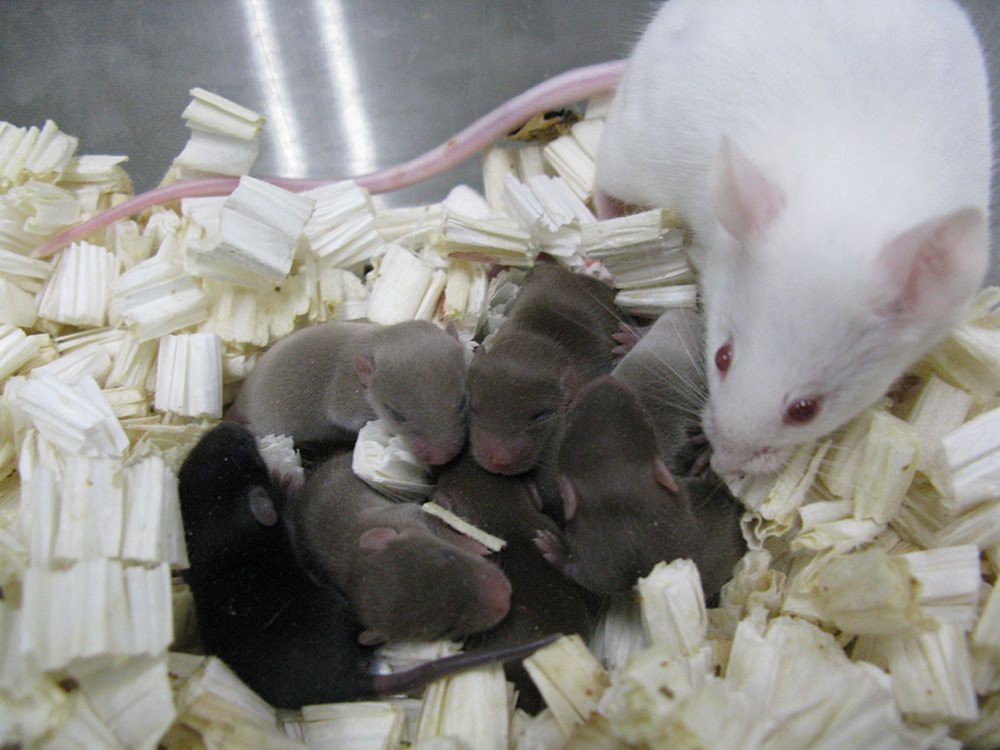
With proposed missions to Mars and plans to establish outposts on the Moon in the coming decades, there are several questions about what effects time spent in space or on other planets could have on the human body. Beyond the normal range of questions concerning the effects of radiation and lower-g on our muscles, bones, and organs, there is also the question of how space travel could impact our ability to reproduce.
Earlier this week – on Monday, May 22nd – a team of Japanese researchers announced findings that could shed light on this question. Using a sample of freeze-dried mouse sperm, the team was able to produce a litter of healthy baby mice. As part of a fertility study, the mouse sperm had spent nine months aboard the International Space Station (between 2013 and 2014). The real question now is, can the same be done for human babies?
The study was led by University of Yamanashi‘s Advanced Biotechnology Center. As she and her colleagues explain in their study – which was recently published in the Proceedings of the National Academy of Sciences – assisted reproductive technology will be needed if humanity ever intends to live in space long-term.
, a student researcher at theAs such, studies that address the effect that living in space could have on human reproduction are needed first. These need to address the impact microgravity (or low-gravity) could have on fertility, human abilities to conceive, and the development of children. And more importantly, they need to deal with one of the greatest hazards of spending time in space – which is the threat posed by solar and cosmic radiation.
To be fair, one need not go far to feel the effects of space radiation. The ISS regularly receives more than 100 times the amount of radiation that Earth’s surface does, which can result in genetic damage if sufficient safeguards are not in place. On other Solar bodies – like Mars and the Moon, which do not have a protective magnetosphere – the situation is similar.
And while the effects of radiation on adults has been studied extensively, the potential damage that could be caused to our offspring has not. How might solar and cosmic radiation affect our ability to reproduce, and how might this radiation affect children when they are still in the womb, and once they are born? Hoping to take the first steps in addressing these questions, Wakayama and her colleagues selected the spermatozoa of mice.
They specifically chose mice since they are a mammalian species that reproduces sexually. As
“So far, only fish or salamanders were examined for reproduction in space. However, mammalian species are very different compared to those species, such as being born from a mother (viviparity). To know whether mammalian reproduction is possible or not, we must use mammalian species for experiments. However, mammalian species such as mice or rats are very sensitive and difficult to take care of by astronauts aboard the ISS, especially for a reproduction study. Therefore, we [have not conducted these studies] until now. We are planning to do more experiments such as the effect of microgravity for embryo development.”
The samples spent nine months aboard the ISS, during which time they were kept at a constant temperature of -95 °C (-139 °F). During launch and recovery, however, they were at room temperature. After retrieval, Wakayama and her team found that the samples had suffered some minor damage,.
“Sperm preserved in space had DNA damage even after only 9 months by space radiation,” said . “However, that damage was not strong and could be repaired when fertilized by oocytes capacity. Therefore, we could obtain normal, healthy offspring. This suggests to me that we must examine the effect when sperm are preserved for longer periods.”
In addition to being reparable, the sperm samples were still able to fertilize mouse embryos (once they were brought back to Earth) and produce mouse offspring, all of which grew to maturity and showed normal fertility levels. They also noted that the fertilization and birth rates were similar to those of control groups, and that only minor genomic differences existed between those and the mouse created using the test sperm.
From all this, they demonstrated that while exposure to space radiation can damage DNA, it need not affect the production of viable offspring (at least within a nine month period). Moreover, the results indicate that human and domestic animals could be produced from space-preserved spermatozoa, which could be mighty useful when it comes to colonizing space and other planets.
As put it, this research builds on fertilization practices already established on Earth, and demonstrated that these same practices could be used in space:
“Our main subject is domestic animal reproduction. In the current situation on the ground, many animals are born from preserves spermatozoa. Especially in Japan, 100% of milk cows were born from preserved sperm due to economic and breeding reasons. Sometimes, sperm that has been stored for more than 10 years was used to produce cows. If humans live in space for many years, then, our results showed that we can eat beefsteak in the space. For that purpose, we did this study. For humans, our finding will probably help infertile couples.”
This research also paves the way for additional tests that would seek to measure the effects of space radiation on ova and the female reproduction system. Not only could these tests tell us a great deal about how time in space could affect female fertility, it could also have serious implications for astronaut safety. As Ulrike Luderer, a professor of medicine at the University of California and one of the co-authors on the paper said in a statement to the AFP:
“These types of exposures can cause early ovarian failure and ovarian cancer, as well as other osteoporosis, cardiovascular disease and neurocognitive diseases like Alzheimer’s. Half the astronauts in the NASA’s new astronaut classes are women. So it is really important to know what chronic health effects there could be for women exposed to long-term deep space radiation.”
However, a lingering issue with these sorts of tests is being able to differentiate between the effects of microgravity and radiation. In the past, research has been conducted that showed how exposure to simulated microgravity can reduce DNA repair capacity and induce DNA damage in humans. Other studies have raised the issue of the interplay between the two, and how further experiments are needed to address the precise impact of each.
In the future, it may be possible to differentiate between the two by placing samples of spermatazoa and ova in a torus that is capable of simulating Earth gravity (1 g). Similarly, shielded modules could be used to isolate the effects of low or even micro-gravity. Beyond that, there will likely be lingering uncertainties until such time as babies are actually born in space, or in a lunar or Martian environment.
And of course, the long-terms impact of reduced gravity and radiation on human evolution remains to be seen. In all likelihood, that won’t become clear for generations to come, and will require multi-generational studies of children born away from Earth to see how they and their progeny differ.
Watch Stars Orbit The Milky Way’s Supermassive Black Hole
When your ordinary citizen learns there’s a supermassive black hole with a mass of 4 million suns sucking on its teeth in the center of the Milky Way galaxy, they might kindly ask exactly how astronomers know this. A perfectly legitimate question. You can tell them that the laws of physics guarantee their existence or that people have been thinking about black holes since 1783. That year, English clergyman John Michell proposed the idea of “dark stars” so massive and gravitationally powerful they could imprison their own light.
Credit: A. Eckart (U. Koeln) & R. Genzel (MPE-Garching), SHARP I, NTT, La Silla Obs., ESO
Michell wasn’t making wild assumptions but taking the idea of gravity to a logical conclusion. Of course, he had no way to prove his assertion. But we do. Astronomers now routinely find bot stellar mass black holes — remnants of the collapse of gas-guzzling supergiant stars — and the supermassive variety in the cores of galaxies that result from multiple black hole mergers over grand intervals of time.
Some of the galactic variety contain hundreds of thousands to billions of solar masses, all of it so to speak “flushed down the toilet” and unavailable to fashion new planets and stars. Famed physicist Stephen Hawking has shown that black holes evaporate over time, returning their energy to the knowable universe from whence they came, though no evidence of the process has yet been found.
So how do we really know a massive, dark object broods at the center of our sparkling Milky Way? Astronomers use radio, X-ray and infrared telescopes to peer into its starry heart and see gas clouds and stars whirling about the center at high rates of speed. Based on those speeds they can calculate the mass of what’s doing the pulling.
In the case of the galaxy M87 located 53.5 million light years away in the Virgo Cluster, those speeds tell us that something with a mass of 3.6 billion suns is concentrated in a space smaller than our Solar System. Oh, and it emits no light! Nothing fits the evidence better than a black hole because nothing that massive can exist in so small a space without collapsing in upon itself to form a black hole. It’s just physics, something that Mr. Scott on Star Trek regularly reminded a panicky Captain Kirk.
So it is with the Milky Way, only our black hole amounts to a piddling 4 million-solar-mass light thief confined within a spherical volume of space some 27 million miles in diameter or just shy of Mercury’s perihelion distance from the Sun. This monster hole resides at the location of Sagittarius A* (pronounced A- star), a bright, compact radio source at galactic center about 26,000 light years away.
Video showing a 14-year-long time lapse of stars orbiting Sgr A*
The time-lapse movie, compiled over 14 years, shows the orbits of several dozen stars within the light year of space centered on Sgr A*. We can clearly see the star moving under the influence of a massive unseen body — the putative supermassive black hole. No observations of Sgr A* in visible light are possible because of multiple veils of interstellar dust that lie across our line of sight. They quench its light to the tune of 25 magnitudes.
Merging black holes (the process look oddly biological!). Credit: SXS
How do these things grow so big in the first place? There are a couple of ideas, but astronomers don’t honestly know for sure. Massive gas clouds around early in the galaxy’s history could have collapsed to form multiple supergiants that evolved into black holes which later then coalesced into one big hole. Or collisions among stars in massive, compact star clusters could have built up stellar giants that evolved into black holes. Later, the clusters sank to the center of the galaxy and merged into a single supermassive black hole.
Whichever you chose, merging of smaller holes may explain its origin.
On a clear spring morning before dawn, you can step out to face the constellation Sagittarius low in the southern sky. When you do, you’re also facing in the direction of our galaxy’s supermassive black hole. Although you cannot see it, does it not still exert a certain tug on your imagination?
How Strong is the Force of Gravity on Earth?
Gravity is a pretty awesome fundamental force. If it wasn’t for the Earth’s comfortable 1 g, which causes objects to fall towards the Earth at a speed of 9.8 m/s², we’d all float off into space. And without it, all us terrestrial species would slowly wither and die as our muscles degenerated, our bones became brittle and weak, and our organs ceased to function properly.
So one can say without exaggerations that gravity is not only a fact of life here on Earth, but a prerequisite for it. However, since human beings seem intent on getting off this rock – escaping the “surly bonds of Earth”, as it were – understanding Earth’s gravity and what it takes to escape it is necessary. So just how strong is Earth’s gravity?
Definition:
To break it down, gravity is a natural phenomena in which all things that possess mass are brought towards one another – i.e. asteroids, planets, stars, galaxies, super clusters, etc. The more mass an object has, the more gravity it will exert on objects around it. The gravitational force of an object is also dependent on distance – i.e. the amount it exerts on an object decreases with increased distance.
Gravity is also one of the four fundamental forces which govern all interactions in nature (along with weak nuclear force, strong nuclear force, and electromagnetism). Of these forces, gravity is the weakest, being approximately 1038 times weaker than the strong nuclear force, 1036 times weaker than the electromagnetic force and 1029 times weaker than the weak nuclear force.
As a consequence, gravity has a negligible influence on matter at the smallest of scales (i.e. subatomic particles). However, at the macroscopic level – that of planets, stars, galaxies, etc. – gravity is the dominant force affecting the interactions of matter. It causes the formation, shape and trajectory of astronomical bodies, and governs astronomical behavior. It also played a major role in the evolution of the early Universe.
It was responsible for matter clumping together to form clouds of gas that underwent gravitational collapse, forming the first stars – which were then drawn together to form the first galaxies. And within individual star systems, it caused dust and gas to coalesce to form the planets. It also governs the orbits of the planets around stars, of moons around planets, the rotation of stars around their galaxy’s center, and the merging of galaxies.
Universal Gravitation and Relativity:
Since energy and mass are equivalent, all forms of energy, including light, also cause gravitation and are under the influence of it. This is consistent with Einstein’s General Theory of Relativity, which remains the best means of describing gravity’s behavior. According to this theory, gravity is not a force, but a consequence of the curvature of spacetime caused by the uneven distribution of mass/energy.
The most extreme example of this curvature of spacetime is a black hole, from which nothing can escape. Black holes are usually the product of a supermassive star that has gone supernova, leaving behind a white dwarf remnant that has so much mass, it’s escape velocity is greater than the speed of light. An increase in gravity also results in gravitational time dilation, where the passage of time occurs more slowly.
For most applications though, gravity is best explained by Newton’s Law of Universal Gravitation, which states that gravity exists as an attraction between two bodies. The strength of this attraction can calculated mathematically, where the attractive force is directly proportional to the product of their masses and inversely proportional to the square of the distance between them.
Earth’s Gravity:
On Earth, gravity gives weight to physical objects and causes the ocean tides. The force of Earth’s gravity is the result of the planets mass and density – 5.97237 × 1024 kg (1.31668×1025 lbs) and 5.514 g/cm3, respectively. This results in Earth having a gravitational strength of 9.8 m/s² close to the surface (also known as 1 g), which naturally decreases the farther away one is from the surface.
In addition, the force of gravity on Earth actually changes depending on where you’re standing on it. The first reason is because the Earth is rotating. This means that the gravity of Earth at the equator is 9.789 m/s2, while the force of gravity at the poles is 9.832 m/s2. In other words, you weigh more at the poles than you do at the equator because of this centripetal force, but only slightly more.
Finally, the force of gravity can change depending on what’s under the Earth beneath you. Higher concentrations of mass, like high-density rocks or minerals can change the force of gravity that you feel. But of course, this amount is too slight to be noticeable. NASA missions have mapped the Earth’s gravity field with incredible accuracy, showing variations in its strength, depending on location.
Gravity also decreases with altitude, since you’re further away from the Earth’s center. The decrease in force from climbing to the top of a mountain is pretty minimal (0.28% less gravity at the top of Mount Everest), but if you’re high enough to reach the International Space Station (ISS), you would experience 90% of the force of gravity you’d feel on the surface.
However, since the station is in a state of free fall (and also in the vacuum of space) objects and astronauts aboard the ISS are capable of floating around. Basically, since everything aboard the station is falling at the same rate towards the Earth, those aboard the ISS have the feeling of being weightless – even though they still weight about 90% of what they would on Earth’s surface.
Earth’s gravity is also responsible for our planet having an “escape velocity” of 11.186 km/s (or 6.951 mi/s). Essentially, this means that a rocket needs to achieve this speed before it can hope to break free of Earth’s gravity and reach space. And with most rocket launches, the majority of their thrust is dedicated to this task alone.
Because of the difference between Earth’s gravity and the gravitational force on other bodies – like the Moon (1.62 m/s²; 0.1654 g) and Mars (3.711 m/s²; 0.376 g) – scientists are uncertain what the effects would be to astronauts who went on long-term missions to these bodies.
While studies have shown that long-duration missions in microgravity (i.e. on the ISS) have a detrimental effect on astronaut health (including loss of bone density, muscle degeneration, damage to organs and to eyesight) no studies have been conducted regarding the effects of lower-gravity environments. But given the multiple proposals made to return to the Moon, and NASA’s proposed “Journey to Mars“, that information should be forthcoming!
As terrestrial beings, we humans are both blessed and cursed by the force of Earth’s gravity. On the one hand, it makes getting into space rather difficult and expensive. On the other, it ensures our health, since our species is the product of billions of years of species evolution that took place in a 1 g environment.
If we ever hope to become a truly space-faring and interplanetary species, we better figure out how we’re going to deal with microgravity and lower-gravity. Otherwise, none of us are likely to get off-world for very long!
We have written many articles about the Earth for Universe Today. Here’s Where Does Gravity Come From?, Who Discovered Gravity?, Why is the Earth Round?, Why Doesn’t the Sun Steal the Moon?, Could We Make Artificial Gravity?, and The “Potsdam Gravity Potato” Shows Variations in Earth’s Gravity.
Want more resources on the Earth? Here’s a link to NASA’s Human Spaceflight page, and here’s NASA’s Visible Earth.
We have also recorded an episode of Astronomy Cast about Earth, as part of our tour through the Solar System – Episode 51: Earth, and Episode 318: Escape Velocity.
Sources: