Since it established orbit around Jupiter in July of 2016, the Juno mission has been sending back vital information about the gas giant’s atmosphere, magnetic field and weather patterns. With every passing orbit – known as perijoves, which take place every 53 days – the probe has revealed more interesting things about Jupiter, which scientists will rely on to learn more about its formation and evolution.
During its latest pass, the probe managed to provide the most detailed look to date of the planet’s interior. In so doing, it learned that Jupiter’s powerful magnetic field is askew, with different patterns in it’s northern and southern hemispheres. These findings were shared on Wednesday. Oct. 18th, at the 48th Meeting of the American Astronomical Society’s Division of Planetary Sciencejs in Provo, Utah.
Ever since astronomers began observing Jupiter with powerful telescopes, they have been aware of its swirling, banded appearance. These colorful stripes of orange, brown and white are the result of Jupiter’s atmospheric composition, which is largely made up of hydrogen and helium but also contains ammonia crystals and compounds that change color when exposed to sunlight (aka. chromofores).
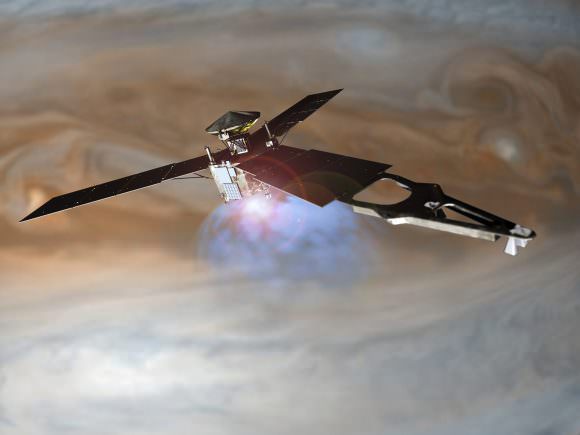
Until now, researchers have been unclear as to whether or not these bands are confined to a shallow layer of the atmosphere or reach deep into the interior of the planet. Answering this question is one of the main goals of the Juno mission, which has been studying Jupiter’s magnetic field to see how it’s interior atmosphere works. Based on the latest results, the Juno team has concluded that hydrogen-rich gas is flowing asymmetrically deep in the planet.
These findings were also presented in a study titled Comparing Jupiter interior structure models to Juno gravity measurements and the role of a dilute core, which appeared in the May 28th issue of Geophysical Research Letters. The study was led by Sean Wahl, a grad student from UC Berkeley, and included members from the Weizmann Institute of Science, the Southwest Research Institute (SwRI), NASA’s Goddard Space Flight Center and the Jet Propulsion Laboratory.
Another interesting find was that Jupiter’s gravity field varies with depth, which indicated that material is flowing as far down as 3,000 km (1,864 mi). Combined with information obtained during previous perijoves, this latest data suggests that Jupiter’s core is small and poorly defined. This flies in the face of previous models of Jupiter, which held that the outer layers are gaseous while the interior ones are made up of metallic hydrogen and a rocky core.
As Tristan Guillot – a planetary scientist at the Observatory of the Côte d’Azur in Nice, France, and a co-author on the study – indicated during the meeting, “This is something that was not expected. We were not sure at all whether we would be able to see that… It’s clear that giant planets have a lot of secrets.”

But of course, more passes and data are needed in order to pinpoint how strong the flow of gases are at various depths, which could resolve the question of how Jupiter’s interior is structured. In the meantime, the Juno scientists are pouring over the probe’s gravity data hoping to see what else it can teach them. For instance, they also want to know how far the Great Red Spot extends into the amotpshere.
This anticyclonic storm, which was first spotted in the 17th century, is Jupiter’s most famous feature. In addition to being large enough to swallow Earth whole – measuring some 16,000 kilometers (10,000 miles) in diameter – wind speeds can reach up to 120 meters per second (432 km/h; 286 mph) at its edges. Already the JunoCam has snapped some very impressive pictures of this storm, and other data has indicated that the storm could run deep.
In fact, on July 10th, 2017, the Juno probe passed withing 9,000 km (5,600 mi) of the Great Red Spot, which took place during its sixth orbit (perijove six) of Jupiter. With it’s suite of eight scientific instruments directed at the storm, the probe obtained readings that indicated that the Great Red Spot could also extend hundreds of kilometers into the interior, or possibly even deeper.
As David Stevenson, a planetary scientist at the California Institute of Technology and a co-author on the study, said during the meeting, “It’s not yet clear that it is so deep it will show up in gravity data. But we’re trying”.
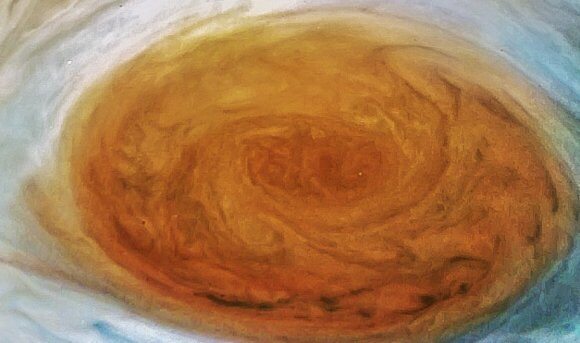
Other big surprises which Juno has revealed since it entered orbit around Jupiter include the clusters of cyclones located at each pole. These were visible to the probe’s instruments in both the visible and infrared wavelengths as it made its first maneuver around the planet, passing from pole to pole. Since Juno is the first space probe in history to orbit the planet this way, these storms were previously unknown to scientists.
In total, Juno spotted eight cyclonic storms around the north pole and five around the south pole. Scientists were especially surprised to see these, since computer modelling suggests that such small storms would not be stable around the poles due to the planet’s swirling polar winds. The answer to this, as indicated during the presentation, may have to do with a concept known as vortex crystals.
As Fachreddin Tabataba-Vakili – a planetary scientist at NASA’s Jet Propulsion Laboratory and a co-author on the study – explained, such crystals are created when small vortices form and persist as the material in which they are embedded continues to flow. This phenomenon has been seen on Earth in the form of rotating superfluids, and Jupiter’s swirling poles may possess similar dynamics.
In the short time that Juno has been operating around Jupiter, it has revealed much about the planet’s atmosphere, interior, magnetic field and internal dynamics. Long after the mission is complete – which will take place in February of 2018 when the probe is crashed into Jupiter’s atmosphere – scientists are likely to be sifting through all the data it obtained, hoping to solve any remaining mysteries from the Solar System’s largest and most massive planet.
Further Reading: Nature