One of my favourite science and engineering facts is that an underground river was frozen to enable the Large Hadron Collider (LHC) to be built! On its completion, it helped to complete the proverbial jigsaw of the Standard Model with is last piece, the Higgs Boson. But that’s about as far as it has got with no other exciting leaps forward in uniting gravity and quantum physics. Plans are now afoot to build a new collider that will be three times longer than the LHC and it will be capable of smashing particles together with significantly more energy.
Continue reading “CERN Wants to Build an Enormous New Atom Smasher: the Future Circular Collider”Anti-Helium Generated in the Large Hadron Collider can Help in the Search for Dark Matter
For decades, astrophysicists have theorized that the majority of matter in our Universe is made up of a mysterious invisible mass known as “Dark Matter” (DM). While scientists have not yet found any direct evidence of this invisible mass or confirmed what it looks like, there are several possible ways we could search for it soon. One theory is that Dark Matter particles could collide and annihilate each other to produce cosmic rays that proliferate throughout our galaxy – similar to how cosmic ray collisions with the interstellar medium (ISM) do.
This theory could be tested soon, thanks to research conducted using the A Large Ion Collider Experiment (ALICE), one of several detector experiments at CERN’s Large Hadron Collider (LHC). ALICE is optimized to study the results from collisions between nuclei that travel very close to the speed of light (ultra-relativistic velocities). According to new research by the ALICE Collaboration, dedicated instruments could detect anti-helium-3 nuclei (the anti-matter counterpart to He3) as they reach Earth’s atmosphere, thus providing evidence for DM.
Continue reading “Anti-Helium Generated in the Large Hadron Collider can Help in the Search for Dark Matter”LHC Scientists Find Three Exotic Particles — and Start Hunting for More
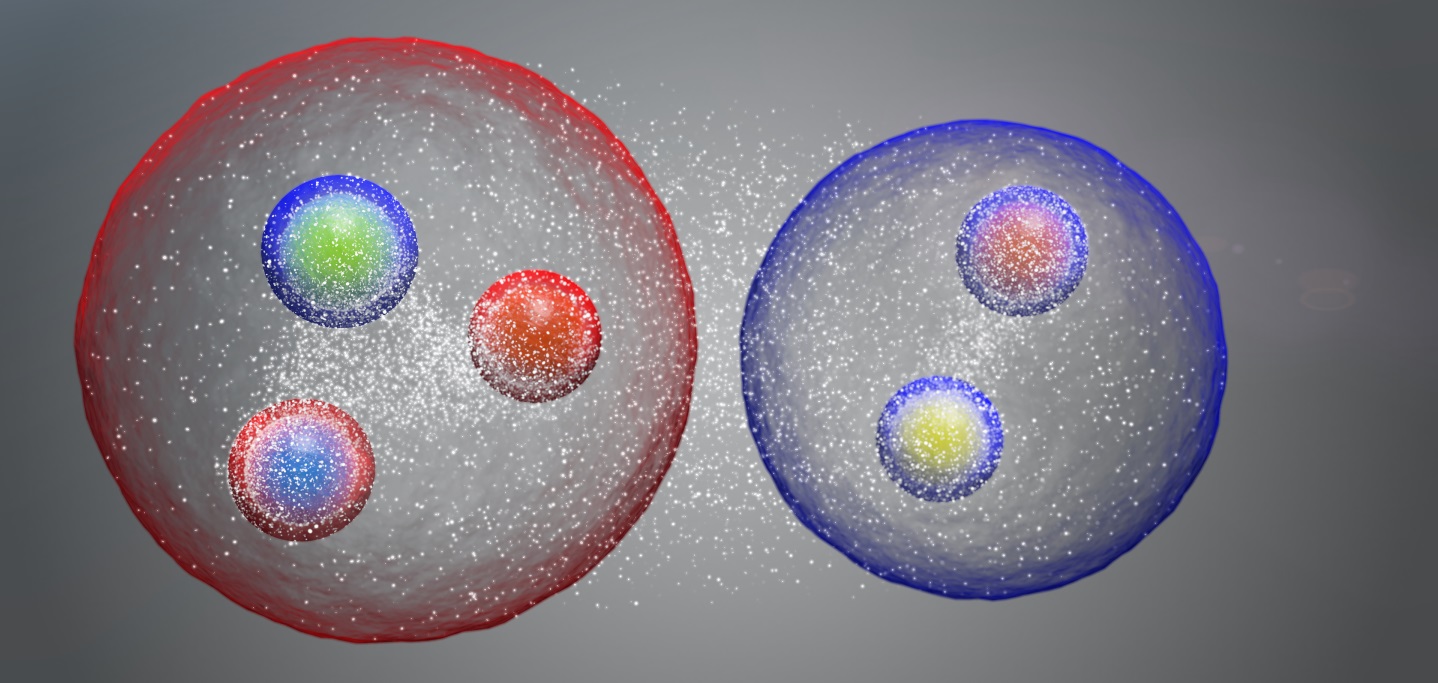
Physicists say they’ve found evidence in data from Europe’s Large Hadron Collider for three never-before-seen combinations of quarks, just as the world’s largest particle-smasher is beginning a new round of high-energy experiments.
The three exotic types of particles — which include two four-quark combinations, known as tetraquarks, plus a five-quark unit called a pentaquark — are totally consistent with the Standard Model, the decades-old theory that describes the structure of atoms.
In contrast, scientists hope that the LHC’s current run will turn up evidence of physics that goes beyond the Standard Model to explain the nature of mysterious phenomena such as dark matter. Such evidence could point to new arrays of subatomic particles, or even extra dimensions in our universe.
Continue reading “LHC Scientists Find Three Exotic Particles — and Start Hunting for More”Large Hadron Collider Restarts, Shooting Protons at Record Energy Levels
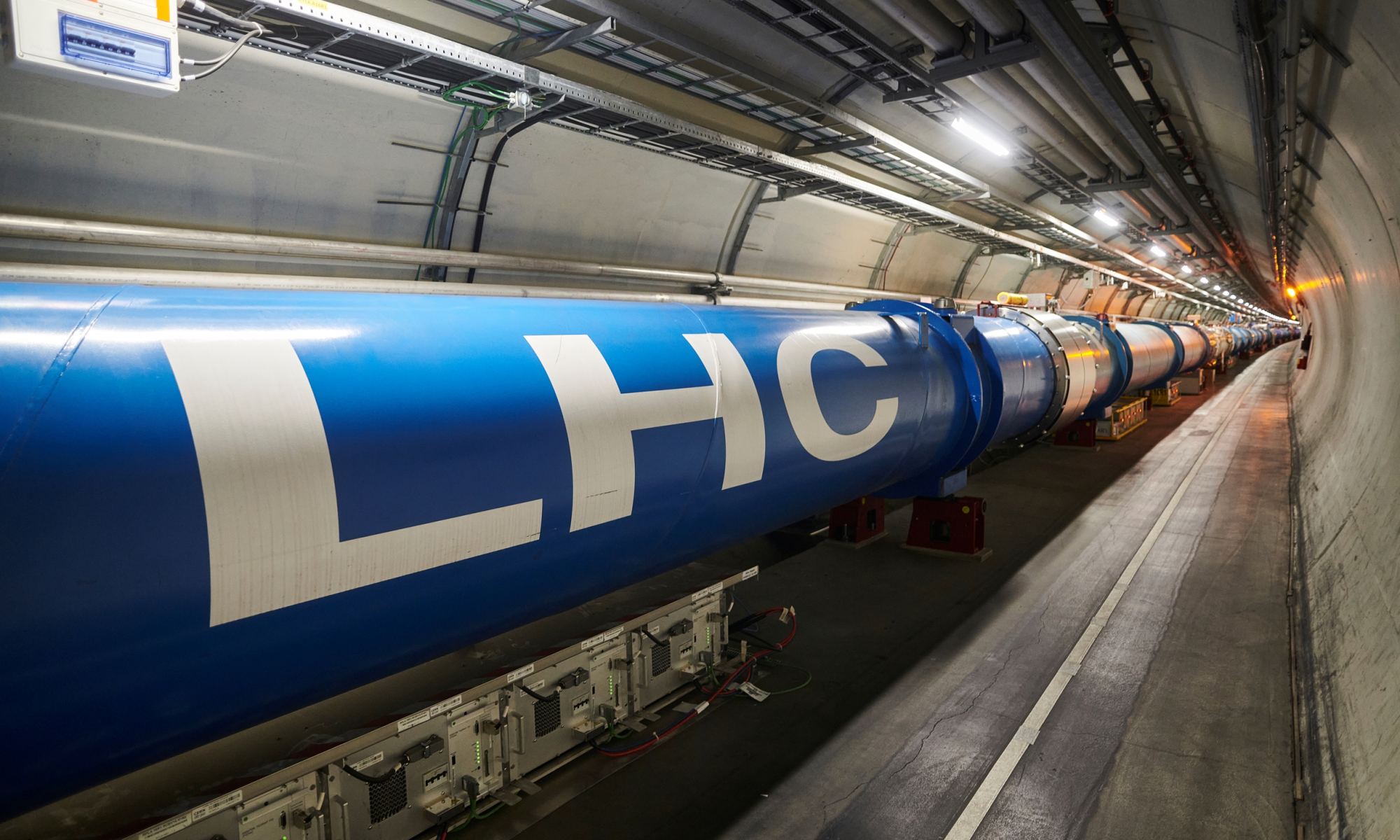
Europe’s Large Hadron Collider has started up its proton beams again at unprecedented energy levels after going through a three-year shutdown for maintenance and upgrades.
It only took a couple of days of tweaking for the pilot streams of protons to reach a record energy level of 6.8 tera electronvolts, or TeV. That exceeds the previous record of 6.5 TeV, which was set by the LHC in 2015 at the start of the particle collider’s second run.
The new level comes “very close to the design energy of the LHC, which is 7 TeV,” Jörg Wenninger, head of the LHC beam operation section and LHC machine coordinator at CERN, said today in a video announcing the milestone.
When the collider at the French-Swiss border resumes honest-to-goodness science operations, probably within a few months, the international LHC team plans to address mysteries that could send theories of physics in new directions.
Continue reading “Large Hadron Collider Restarts, Shooting Protons at Record Energy Levels”
CERN is Planning to Build a Much Larger Particle Collider. Much, Much, Larger.
CERN, the European Organization for Nuclear Research, wants to build a particle collider that will dwarf the Large Hadron Collider (LHC). The LHC has made important discoveries, and planned upgrades to its power ensures it will keep working on physics problems into the future. But eventually, it won’t be enough to unlock the secrets of physics. Eventually, we’ll need something larger and more powerful.
Enter the Future Circular Collider (FCC.) The FCC will exceed the LHC in power by an order of magnitude. On January 15th, the FCC collaboration released its Conceptual Design Report (CDR) that lays out the options for CERN’s Future Circular Collider.
Continue reading “CERN is Planning to Build a Much Larger Particle Collider. Much, Much, Larger.”The Large Hadron Collider has been Shut Down, and Will Stay Down for Two Years While they Perform Major Upgrades
The Large Hadron Collider (LHC) is getting a big boost to its performance. Unfortunately, for fans of ground-breaking physics, the whole thing has to be shut down for two years while the work is done. But once it’s back up and running, its enhanced capabilities will make it even more powerful.
Continue reading “The Large Hadron Collider has been Shut Down, and Will Stay Down for Two Years While they Perform Major Upgrades”
Another Strange Discovery From LHC That Nobody Understands
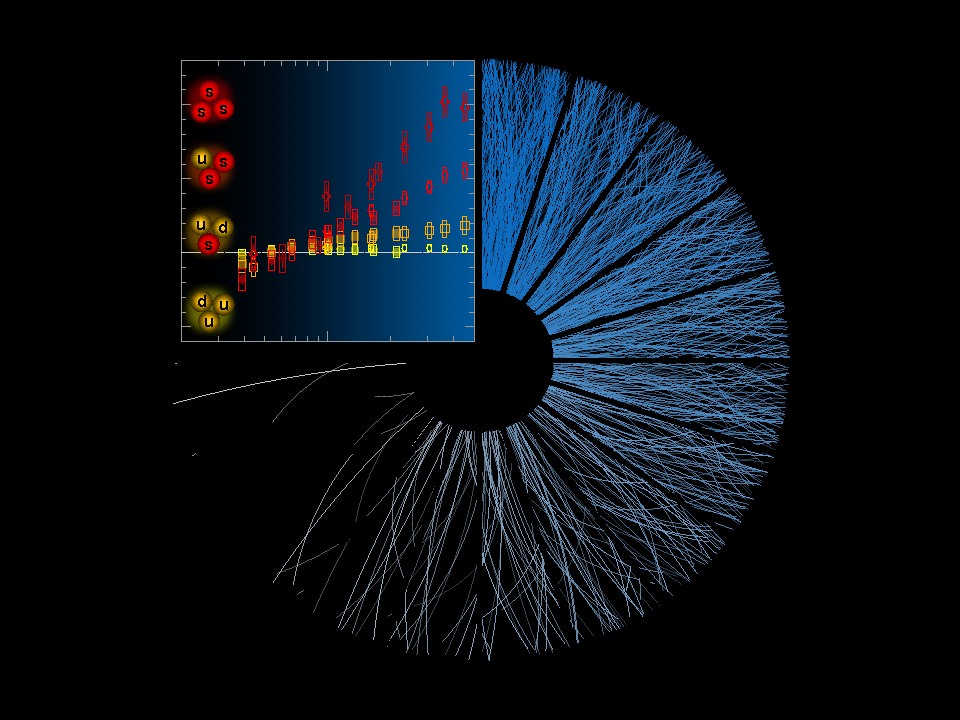
There are some strange results being announced in the physics world lately. A fluid with a negative effective mass, and the discovery of five new particles, are all challenging our understanding of the universe.
New results from ALICE (A Large Ion Collider Experiment) are adding to the strangeness.
ALICE is a detector on the Large Hadron Collider (LHC). It’s one of seven detectors, and ALICE’s role is to “study the physics of strongly interacting matter at extreme energy densities, where a phase of matter called quark-gluon plasma forms,” according to the CERN website. Quark-gluon plasma is a state of matter that existed only a few millionths of a second after the Big Bang.
In what we might call normal matter—that is the familiar atoms that we all learn about in high school—protons and neutrons are made up of quarks. Those quarks are held together by other particles called gluons. (“Glue-ons,” get it?) In a state known as confinement, these quarks and gluons are permanently bound together. In fact, quarks have never been observed in isolation.
The LHC is used to collide particles together at extremely high speeds, creating temperatures that can be 100,000 times hotter than the center of our Sun. In new results just released from CERN, lead ions were collided, and the resulting extreme conditions come close to replicating the state of the Universe those few millionths of a second after the Big Bang.
In those extreme temperatures, the state of confinement was broken, and the quarks and gluons were released, and formed quark-gluon plasma.
So far, this is pretty well understood. But in these new results, something additional happened. There was increased production of what are called “strange hadrons.” Strange hadrons themselves are well-known particles. They have names like Kaon, Lambda, Xi and Omega. They’re called strange hadrons because they each have one “strange quark.”
If all of this seems a little murky, here’s the dinger: Strange hadrons may be well-known particles, because they’ve been observed in collisions between heavy nuclei. But they haven’t been observed in collisions between protons.
“Being able to isolate the quark-gluon-plasma-like phenomena in a smaller and simpler system…opens up an entirely new dimension for the study of the properties of the fundamental state that our universe emerged from.” – Federico Antinori, Spokesperson of the ALICE collaboration.
“We are very excited about this discovery,” said Federico Antinori, Spokesperson of the ALICE collaboration. “We are again learning a lot about this primordial state of matter. Being able to isolate the quark-gluon-plasma-like phenomena in a smaller and simpler system, such as the collision between two protons, opens up an entirely new dimension for the study of the properties of the fundamental state that our universe emerged from.”
Enhanced Strangeness?
The creation of quark-gluon plasma at CERN provides physicists an opportunity to study the strong interaction. The strong interaction is also known as the strong force, one of the four fundamental forces in the Universe, and the one that binds quarks into protons and neutrons. It’s also an opportunity to study something else: the increased production of strange hadrons.
In a delicious turn of phrase, CERN calls this phenomenon “enhanced strangeness production.” (Somebody at CERN has a flair for language.)
Enhanced strangeness production from quark-gluon plasma was predicted in the 1980s, and was observed in the 1990s at CERN’s Super Proton Synchrotron. The ALICE experiment at the LHC is giving physicists their best opportunity yet to study how proton-proton collisions can have enhanced strangeness production in the same way that heavy ion collisions can.
According to the press release announcing these results, “Studying these processes more precisely will be key to better understand the microscopic mechanisms of the quark-gluon plasma and the collective behaviour of particles in small systems.”
I couldn’t have said it better myself.
Large Hadron Collider Discovers 5 New Gluelike Particles
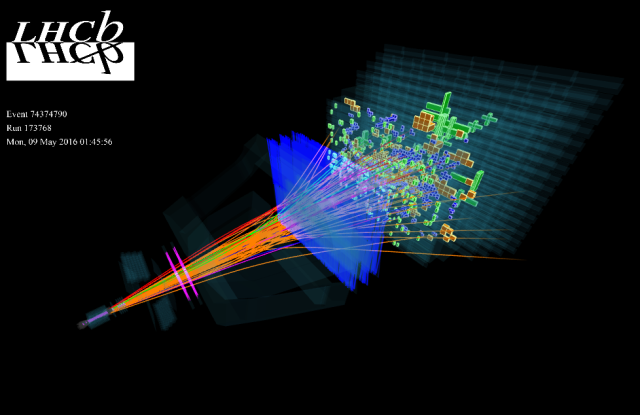
Since it began its second operational run in 2015, the Large Hadron Collider has been doing some pretty interesting things. For example, starting in 2016, researchers at CERN began using the collide to conduct the Large Hadron Collider beauty experiment (LHCb). This is investigation seeks to determine what it is that took place after the Big Bang so that matter was able to survive and create the Universe that we know today.
In the past few months, the experiment has yielded some impressive results, such as the measurement of a very rare form of particle decay and evidence of a new manifestation of matter-antimatter asymmetry. And most recently, the researchers behind LHCb have announced the discovery of a new system of five particles, all of which were observed in a single analysis.
According to the research paper, which appeared in arXiv on March 14th, 2017, the particles that were detected were excited states of what is known as a “Omega-c-zero” baryon. Like other particles of its kind, the Omega-c-zero is made up of three quarks – two of which are “strange” while the third is a “charm” quark. The existence of this baryon was confirmed in 1994. Since then, researchers at CERN have sought to determine if there were heavier versions.
And now, thanks to the LHCb experiment, it appears that they have found them. The key was to examine the trajectories and the energy left in the detector by particles in their final configuration and trace them back to their original state. Basically, Omega-c-zero particles decay via the strong force into another type of baryon (Xi-c-plus) and then via the weak force into protons, kaons, and pions.
From this, the researchers were able to determine that what they were seeing were Omega-c-zero particles at different energy states (i.e. of different sizes and masses). Expressed in megaelectronvolts (MeV), these particles have masses of 3000, 3050, 3066, 3090 and 3119 MeV, respectively. This discovery was rather unique, since it involved the detection of five higher energy states of a particle at the same time.
This was made possible thanks to the specialized capabilities of the LHCb detector and the large dataset that was accumulated from the first and second runs of the LHC – which ran from 2009 to 2013, and since 2015, respectively. Armed with the right equipment and experience, the researchers were able to identify the particles with an overwhelming level of certainty, ruling out the possibility that it was a statistical fluke in the data.
The discovery is also expected to shed light on some of the deeper mysteries of subatomic particles, like how the three constituent quarks are bound inside a baryon by the “strong force” – i.e. the fundamental force that is responsible for holding the insides of atoms together. Another mystery that this could help resolve in the correlation between different quark states.
As Dr Greig Cowan – a researcher from the University of Edinburgh who works on the LHCb experiment at Cern’s LHC – explained in an interview with the BBC:
“This is a striking discovery that will shed light on how quarks bind together. It may have implications not only to better understand protons and neutrons, but also more exotic multi-quark states, such as pentaquarks and tetraquarks.“
The next step will be to determine the quantum numbers of these new particles (the numbers used to identify the properties of a specific particle) as well as determining their theoretical significance. Since it came online, the LHC has been helping to confirm the Standard Model of particle physics, as well as reaching beyond it to explore the greater unknowns of how the Universe came to be, and how the fundamental forces that govern it fit together.
In the end, the discovery of these five new particles could be a crucial step along the road towards a Theory of Everything (ToE), or just another piece in the very big puzzle that is our existence. Stay tuned to see which!
What is the CERN Particle Accelerator?
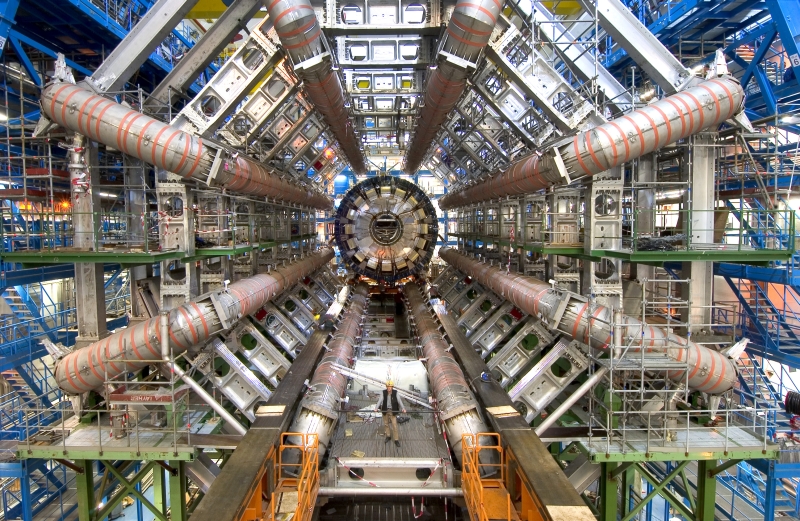
What if it were possible to observe the fundamental building blocks upon which the Universe is based? Not a problem! All you would need is a massive particle accelerator, an underground facility large enough to cross a border between two countries, and the ability to accelerate particles to the point where they annihilate each other – releasing energy and mass which you could then observe with a series of special monitors.
Well, as luck would have it, such a facility already exists, and is known as the CERN Large Hardron Collider (LHC), also known as the CERN Particle Accelerator. Measuring roughly 27 kilometers in circumference and located deep beneath the surface near Geneva, Switzerland, it is the largest particle accelerator in the world. And since CERN flipped the switch, the LHC has shed some serious light on some deeper mysteries of the Universe.
Purpose:
Colliders, by definition, are a type of a particle accelerator that rely on two directed beams of particles. Particles are accelerated in these instruments to very high kinetic energies and then made to collide with each other. The byproducts of these collisions are then analyzed by scientists in order ascertain the structure of the subatomic world and the laws which govern it.
The purpose of colliders is to simulate the kind of high-energy collisions to produce particle byproducts that would otherwise not exist in nature. What’s more, these sorts of particle byproducts decay after very short period of time, and are are therefor difficult or near-impossible to study under normal conditions.
The term hadron refers to composite particles composed of quarks that are held together by the strong nuclear force, one of the four forces governing particle interaction (the others being weak nuclear force, electromagnetism and gravity). The best-known hadrons are baryons – protons and neutrons – but also include mesons and unstable particles composed of one quark and one antiquark.
Design:
The LHC operates by accelerating two beams of “hadrons” – either protons or lead ions – in opposite directions around its circular apparatus. The hadrons then collide after they’ve achieved very high levels of energy, and the resulting particles are analyzed and studied. It is the largest high-energy accelerator in the world, measuring 27 km (17 mi) in circumference and at a depth of 50 to 175 m (164 to 574 ft).
The tunnel which houses the collider is 3.8-meters (12 ft) wide, and was previously used to house the Large Electron-Positron Collider (which operated between 1989 and 2000). This tunnel contains two adjacent parallel beamlines that intersect at four points, each containing a beam that travels in opposite directions around the ring. The beam is controlled by 1,232 dipole magnets while 392 quadrupole magnets are used to keep the beams focused.
About 10,000 superconducting magnets are used in total, which are kept at an operational temperature of -271.25 °C (-456.25 °F) – which is just shy of absolute zero – by approximately 96 tonnes of liquid helium-4. This also makes the LHC the largest cryogenic facility in the world.
When conducting proton collisions, the process begins with the linear particle accelerator (LINAC 2). After the LINAC 2 increases the energy of the protons, these particles are then injected into the Proton Synchrotron Booster (PSB), which accelerates them to high speeds.
They are then injected into the Proton Synchrotron (PS), and then onto the Super Proton Synchrtron (SPS), where they are sped up even further before being injected into the main accelerator. Once there, the proton bunches are accumulated and accelerated to their peak energy over a period of 20 minutes. Last, they are circulated for a period of 5 to 24 hours, during which time collisions occur at the four intersection points.
During shorter running periods, heavy-ion collisions (typically lead ions) are included the program. The lead ions are first accelerated by the linear accelerator LINAC 3, and the Low Energy Ion Ring (LEIR) is used as an ion storage and cooler unit. The ions are then further accelerated by the PS and SPS before being injected into LHC ring.
While protons and lead ions are being collided, seven detectors are used to scan for their byproducts. These include the A Toroidal LHC ApparatuS (ATLAS) experiment and the Compact Muon Solenoid (CMS), which are both general purpose detectors designed to see many different types of subatomic particles.
Then there are the more specific A Large Ion Collider Experiment (ALICE) and Large Hadron Collider beauty (LHCb) detectors. Whereas ALICE is a heavy-ion detector that studies strongly-interacting matter at extreme energy densities, the LHCb records the decay of particles and attempts to filter b and anti-b quarks from the products of their decay.
Then there are the three small and highly-specialized detectors – the TOTal Elastic and diffractive cross section Measurement (TOTEM) experiment, which measures total cross section, elastic scattering, and diffractive processes; the Monopole & Exotics Detector (MoEDAL), which searches magnetic monopoles or massive (pseudo-)stable charged particles; and the Large Hadron Collider forward (LHCf) that monitor for astroparticles (aka. cosmic rays).
History of Operation:
CERN, which stands for Conseil Européen pour la Recherche Nucléaire (or European Council for Nuclear Research in English) was established on Sept 29th, 1954, by twelve western European signatory nations. The council’s main purpose was to oversee the creation of a particle physics laboratory in Geneva where nuclear studies would be conducted.
Soon after its creation, the laboratory went beyond this and began conducting high-energy physics research as well. It has also grown to include twenty European member states: France, Switzerland, Germany, Belgium, the Netherlands, Denmark, Norway, Sweden, Finland, Spain, Portugal, Greece, Italy, the UK, Poland, Hungary, the Czech Republic, Slovakia, Bulgaria and Israel.
Construction of the LHC was approved in 1995 and was initially intended to be completed by 2005. However, cost overruns, budget cuts, and various engineering difficulties pushed the completion date to April of 2007. The LHC first went online on September 10th, 2008, but initial testing was delayed for 14 months following an accident that caused extensive damage to many of the collider’s key components (such as the superconducting magnets).
On November 20th, 2009, the LHC was brought back online and its First Run ran from 2010 to 2013. During this run, it collided two opposing particle beams of protons and lead nuclei at energies of 4 teraelectronvolts (4 TeV) and 2.76 TeV per nucleon, respectively. The main purpose of the LHC is to recreate conditions just after the Big Bang when collisions between high-energy particles was taking place.
Major Discoveries:
During its First Run, the LHCs discoveries included a particle thought to be the long sought-after Higgs Boson, which was announced on July 4th, 2012. This particle, which gives other particles mass, is a key part of the Standard Model of physics. Due to its high mass and elusive nature, the existence of this particle was based solely in theory and had never been previously observed.
The discovery of the Higgs Boson and the ongoing operation of the LHC has also allowed researchers to investigate physics beyond the Standard Model. This has included tests concerning supersymmetry theory. The results show that certain types of particle decay are less common than some forms of supersymmetry predict, but could still match the predictions of other versions of supersymmetry theory.
In May of 2011, it was reported that quark–gluon plasma (theoretically, the densest matter besides black holes) had been created in the LHC. On November 19th, 2014, the LHCb experiment announced the discovery of two new heavy subatomic particles, both of which were baryons composed of one bottom, one down, and one strange quark. The LHCb collaboration also observed multiple exotic hadrons during the first run, possibly pentaquarks or tetraquarks.
Since 2015, the LHC has been conducting its Second Run. In that time, it has been dedicated to confirming the detection of the Higgs Boson, and making further investigations into supersymmetry theory and the existence of exotic particles at higher-energy levels.
In the coming years, the LHC is scheduled for a series of upgrades to ensure that it does not suffer from diminished returns. In 2017-18, the LHC is scheduled to undergo an upgrade that will increase its collision energy to 14 TeV. In addition, after 2022, the ATLAS detector is to receive an upgrade designed to increase the likelihood of it detecting rare processes, known as the High Luminosity LHC.
The collaborative research effort known as the LHC Accelerator Research Program (LARP) is currently conducting research into how to upgrade the LHC further. Foremost among these are increases in the beam current and the modification of the two high-luminosity interaction regions, and the ATLAS and CMS detectors.
Who knows what the LHC will discover between now and the day when they finally turn the power off? With luck, it will shed more light on the deeper mysteries of the Universe, which could include the deep structure of space and time, the intersection of quantum mechanics and general relativity, the relationship between matter and antimatter, and the existence of “Dark Matter”.
We have written many articles about CERN and the LHC for Universe Today. Here’s What is the Higgs Boson?, The Hype Machine Deflates After CERN Data Shows No New Particle, BICEP2 All Over Again? Researchers Place Higgs Boson Discovery in Doubt, Two New Subatomic Particles Found, Is a New Particle about to be Announced?, Physicists Maybe, Just Maybe, Confirm the Possible Discovery of 5th Force of Nature.
If you’d like more info on the Large Hadron Collider, check out the LHC Homepage, and here’s a link to the CERN website.
Astronomy Cast also has some episodes on the subject. Listen here, Episode 69: The Large Hadron Collider and The Search for the Higgs Boson and Episode 392: The Standard Model – Intro.
Sources:
Some Humans Just Sacrificed Their Jobs At CERN
Yes, despite what some people were clearly meant to believe, jobs are about the only thing being sacrificed at CERN recently. After a strange video depicting what was meant to look like a human sacrifice on its Geneva campus went viral, the European Organization for Nuclear Research (CERN) launched an official investigation to get to the bottom of it.
And while the video was quickly determined to be a prank – no doubt to mess with all those who think that CERN is evil and the Large Hadron Collider (LHC) is a “tool of the devil” – it has raised concerns about security on CERN campuses, not to mention the questionable senses of humor of some of its staff!
The video, which began circulating earlier this week, featured some disturbing imagery. Within the main square of CERN’s Geneva campus – which is home to the LHC- several figures appear to be reenacting an occult ceremony. They are seen wearing black cloaks and performing rites in front of a statue of the Hindu deity Shiva – which is on permanent display at the complex.
The scene climaxes with the staged stabbing of a woman, and then ends with the one filming the scene (who appears to be recording everything from a hidden location) uttering some expletives and running off. In response, the European Organization for Nuclear Research issued a statement, claiming they would be investigating.
They also stressed that they considered this to be an “internal matter”. So while the Geneva police were aware of the incident, they will not be formally involved in the investigation. In response to a request for comment from the Agency France-Presse (AFP), a CERN spokewoman replied via email:
“These scenes were filmed on our premises but without official permission or knowledge. CERN does not condone this type of spoof, which can give rise to misunderstandings about the scientific nature of our work.”
According to this same spokeswoman, the people conducting the reenactment were likely staff. While they are not able to confirm the identities of those in the video, CERN’s security measures require that those working on their premises, of have access to their facilities, have official IDs.
“CERN IDs are checked systematically at each entry to the CERN site whether it is night or day,” she said. “CERN welcomes every year thousands of scientific users from all over the world and sometimes some of them let their humor go too far. This is what happened on this occasion.”
The statue used for the prank was none other than the Nataraja – a depicition of Shiva as the cosmic dancer – which is on permanent display at CERN. The statue was a gift issued by the Indian government in 2004 to celebrate the country’s long-standing relationship with the research facility.
Needless to say, there’s likely to be some hell to pay once the prankster’s are identified. While the prank does seem to have a sense of irony to it – as if its specifically mocking tho conspiracy theorists who think evil things go on there – the last thing CERN wants is negative publicity, or people conducting pranks that involve sacred artwork!
If you haven’t seen the footage, be sure to check out this snippet from NewsBeatSocial below:
Further Reading: The Gaurdian