At least once, you’ve looked up at the night sky and asked the same longstanding question we’ve all asked at least once, “Are we alone?” With all those points of light out there, we can’t be the only intelligent beings in the universe, right? There must be at least one technological civilization aside from us in the great vastness that we call the cosmos.
Continue reading “What if we’re truly alone?”Will Triton finally answer, ‘Are we alone?’

We recently examined how and why Saturn’s icy moon, Enceladus, could answer the longstanding question: Are we alone? With its interior ocean and geysers of water ice that shoot out tens of kilometers into space that allegedly contains the ingredients for life, this small moon could be a prime target for future astrobiology missions. But Enceladus isn’t the only location in our solar system with active geysers, as another small moon near the edge of the solar system shares similar characteristics, as well. This is Neptune’s largest moon, Triton, which has been visited only once by NASA’s Voyager 2 in 1989. But are Triton’s geysers the only characteristics that make it a good target for astrobiology and finding life beyond Earth?
Continue reading “Will Triton finally answer, ‘Are we alone?’”Searching for Life on Highly Eccentric Exoplanets
When we think about finding life beyond Earth, especially on exoplanets, we immediately want to search for the next Earth, or Earth 2.0. We want an exoplanet that orbits a star firmly in its habitable zone (HZ) with vast oceans of liquid water, and plenty of land to go around. An exoplanet like that most certainly has life, right? But what if we’re looking in the wrong places? What if we find life on exoplanets that don’t possess the aforementioned characteristics, i.e., Earth 2.0?
Continue reading “Searching for Life on Highly Eccentric Exoplanets”Another Reason Red Dwarfs Might Be Bad for Life: No Asteroid Belts
In a recent study accepted to The Astrophysical Journal Letters, a team of researchers at the University of Nevada, Las Vegas (UNLV) investigated the potential for life on exoplanets orbiting M-dwarf stars, also known as red dwarfs, which are both smaller and cooler than our own Sun and is currently open for debate for their potential for life on their orbiting planetary bodies. The study examines how a lack of an asteroid belt might indicate a less likelihood for life on terrestrial worlds.
Continue reading “Another Reason Red Dwarfs Might Be Bad for Life: No Asteroid Belts”Will Europa finally answer, ‘Are we alone?’
While NASA’s much-lauded Space Launch System stands ready for its maiden flight later this month with the goal of sending astronauts back to the Moon in the next few years, our gazes once again turn to the stars as we continue to ask the question that has plagued humankind since time immemorial: Are we alone? While there are several solar system locales that we can choose from to conduct our search for life beyond Earth, to include Mars and Saturn’s moons, Titan and Enceladus, one planetary body orbiting the largest planet in the solar system has peaked the interest of scientists since the 1970s.
Continue reading “Will Europa finally answer, ‘Are we alone?’”Mars Rovers Will Need to Dig Deeper If They Want to Find Evidence of Life
The search for life—even ancient life—on Mars is trickier than we thought. In a recent study published in the journal Astrobiology, researchers have determined that NASA’s Mars Perseverance (Percy) Rover will have to dig two meters (6.6 feet) beneath the Martian surface in order to find traces of ancient life. This is because the surface of Mars is constantly bombarded with extreme levels of solar radiation that scientists hypothesize would quickly degrade small molecules such as amino acids. The reason for this extreme level of radiation is due to the absence of a magnetic field, which scientists believe was stripped away billions of years ago when the planet’s liquid outer core ceased to produce the dynamo that created the field.
Continue reading “Mars Rovers Will Need to Dig Deeper If They Want to Find Evidence of Life”Life Could be Common Across the Universe, Just Not in Our Region
The building blocks of life can, and did, spontaneously assemble under the right conditions. That’s called spontaneous generation, or abiogenesis. Of course, many of the details remain hidden to us, and we just don’t know exactly how it all happened. Or how frequently it could happen.
Continue reading “Life Could be Common Across the Universe, Just Not in Our Region”Galactic Panspermia: Interstellar Dust Could Transport Life from Star to Star
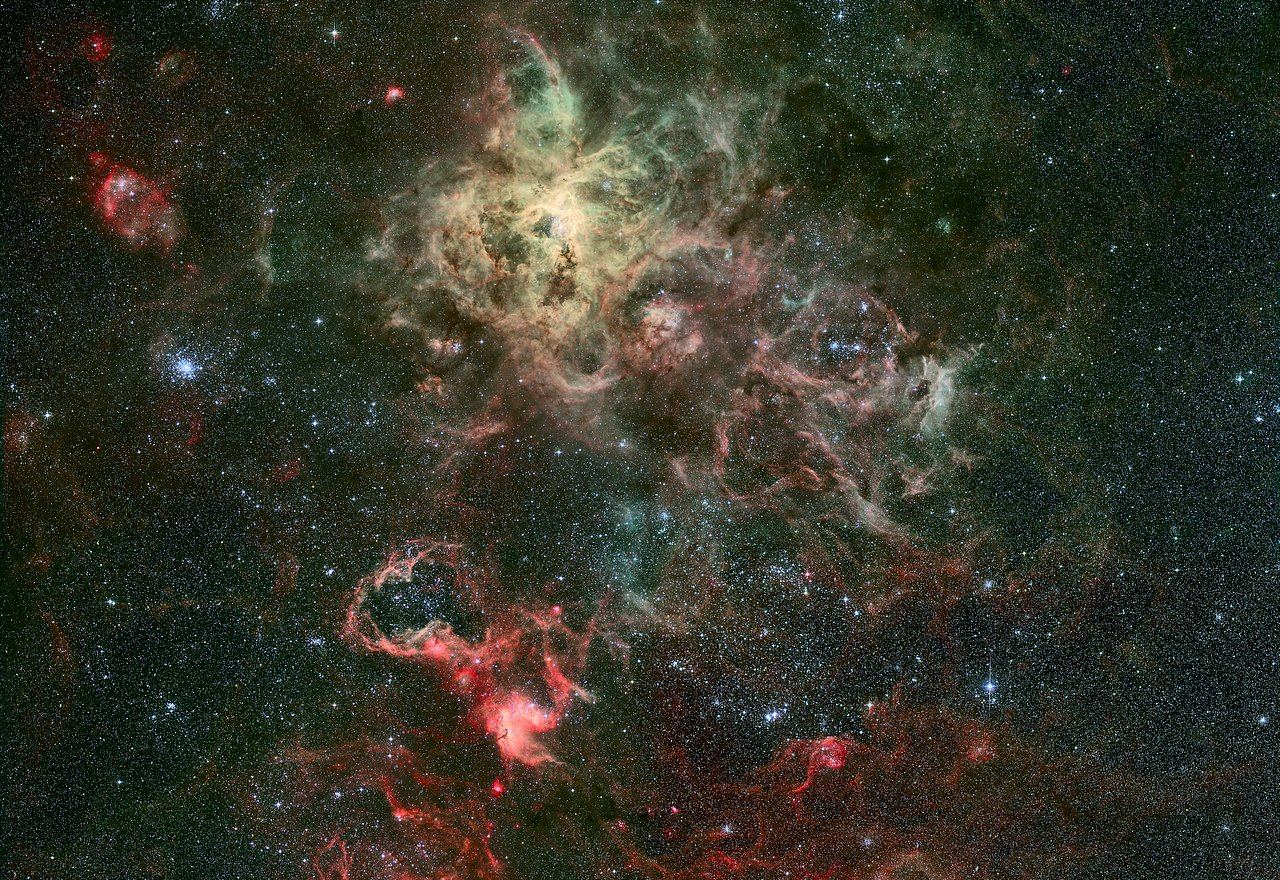
The theory of Panspermia states that life exists through the cosmos, and is distributed between planets, stars and even galaxies by asteroids, comets, meteors and planetoids. In this respect, life began on Earth about 4 billion years ago after microorganisms hitching a ride on space rocks landed on the surface. Over the years, considerable research has been devoted towards demonstrating that the various aspects of this theory work.
The latest comes from the University of Edinburgh, where Professor Arjun Berera offers another possible method for the transport of life-bearing molecules. According to his recent study, space dust that periodically comes into contact with Earth’s atmosphere could be what brought life to our world billions of years ago. If true, this same mechanism could be responsible for the distribution of life throughout the Universe.
For the sake of his study, which was recently published in Astrobiology under the title “Space Dust Collisions as a Planetary Escape Mechanism“, Prof. Berera examined the possibility that space dust could facilitate the escape of particles from Earth’s atmosphere. These include molecules that indicate the presence of life on Earth (aka. biosignatures), but also microbial life and molecules that are essential to life.
Fast-moving flows of interplanetary dust impact our atmosphere on a regular basis, at a rate of about 100,000 kg (110 tons) a day. This dust ranges in mass from 10-18 to 1 gram, and can reach speeds of 10 to 70 km/s (6.21 to 43.49 mps). As a result, this dust is capable of impacting Earth with enough energy to knock molecules out of the atmosphere and into space.
These molecules would consist largely of those that are present in the thermosphere. At this level, those particles would consist largely of chemically disassociated elements, such as molecular nitrogen and oxygen. But even at this high altitude, larger particles – such as those that are capable of harboring bacteria or organic molecules – have also been known to exist. As Dr. Berera states in his study:
“For particles that form the thermosphere or above or reach there from the ground, if they collide with this space dust, they can be displaced, altered in form or carried off by incoming space dust. This may have consequences for weather and wind, but most intriguing and the focus of this paper, is the possibility that such collisions can give particles in the atmosphere the necessary escape velocity and upward trajectory to escape Earth’s gravity.”
Of course, the process of molecules escaping our atmosphere presents certain difficulties. For starters, it requires that there be enough upward force that can accelerate these particles to escape velocity speeds. Second, if these particle are accelerated from too low an altitude (i.e. in the stratosphere or below), the atmospheric density will be high enough to create drag forces that will slow the upward-moving particles.
In addition, as a result of their fast upward travel, these particle would undergo immense heating to the point of evaporation. So while wind, lighting, volcanoes, etc. would be capable of imparting huge forces at lower altitudes, they would not be able to accelerate intact particles to the point where they could achieve escape velocity. On the other hand, in the upper part of the mesosphere and thermosphere, particles would not suffer much drag or heating.
As such, Berera concludes that only atoms and molecules that are already found in the higher atmosphere could be propelled into space by space dust collisions. The mechanism for propelling them there would likely consist of a double state approach, whereby they are first hurled into the lower thermosphere or higher by some mechanism and then propelled even harder by fast space dust collision.
After calculating the speed at which space dust impacts our atmosphere, Berera determined that molecules that exist at an altitude of 150 km (93 mi) or higher above Earth’s surface would be knocked beyond the limit of Earth’s gravity. These molecules would then be in near-Earth space, where they could be picked up by passing objects such as comets, asteroid or other Near-Earth Objects (NEO) and carried to other planets.
Naturally, this raises another all-important question, which is whether or not these organisms could survive in space. But as Berera notes, previous studies have borne out the ability of microbes to survive in space:
“Should some microbial particles manage the perilous journey upward and out of the Earth’s gravity, the question remains how well they will survive in the harsh environment of space. Bacterial spores have been left on the exterior of the International Space Station at altitude ~400km, in a near vacuum environment of space, where there is nearly no water, considerable radiation, and with temperatures ranging from 332K on the sun side to 252K on the shadow side, and have survived 1.5 years.”
Another thing Berera considers is the strange case of tardigrades, the eight-legged micro-animals that are also known as “water bears”. Previous experiments have shown that this species is capable of surviving in space, being both strongly resistant to radiation and desiccation. So it is possible that such organisms, if they were knocked out of Earth’s upper atmosphere, could survive long enough to hitch a ride to another planet
“The proposition that space dust collisions could propel organisms over enormous distances between planets raises some exciting prospects of how life and the atmospheres of planets originated. The streaming of fast space dust is found throughout planetary systems and could be a common factor in proliferating life.”
In addition to offering a fresh take on Panspermia, Berera’s study is also significant when it comes to the study of how life evolved on Earth. If biological molecules and bacteria have been escaping Earth’s atmosphere continuously over the course of its existence, then this would suggest that it could still be floating out in the Solar System, possibly within comets and asteroids.
These biological samples, if they could be accessed and studied, would serve as a timeline for the evolution of microbial life on Earth. It’s also possible that Earth-borne bacteria survive today on other planets, possibly on Mars or other bodies where they locked away in permafrost or ice. These colonies would basically be time capsules, containing preserved life that could date back billions of years.
Further Reading: University of Edinburgh, Astrobiology
What Did Cassini Teach Us?
Ask me my favorite object in the Solar System, especially to see through a telescope, and my answer is always the same: Saturn.
Saturn is this crazy, ringed world, different than any other place we’ve ever seen. And in a small telescope, you can really see the ball of the planet, you can see its rings. It’s one thing to see a world like this from afar, a tiny jumping image in a telescope. To really appreciate and understand a place like Saturn, you’ve got to visit.
And thanks to NASA’s Cassini spacecraft, that’s just what we’ve been doing for the last 13 years. Take a good close look at this amazing ringed planet and its moons, and studying it from every angle.
Throughout this article, I’m going to regale you with the amazing discoveries made by Cassini at Saturn. What it taught us, and what new mysteries it uncovered.
NASA’s Cassini spacecraft was launched from Earth on October 15, 1997. Instead of taking the direct route, it made multiple flybys of Venus, a flyby of Earth and a flyby of Jupiter. Each one of these close encounters boosted Cassini’s velocity, allowing it to make the journey with less escape velocity from Earth.
It arrived at Saturn on July 1st, 2004 and began its science operations shortly after that. The primary mission lasted 4 years, and then NASA extended its mission two more times. The first ending in 2010, and the second due to end in 2017. But more on that later.
Before Cassini, we only had flybys of Saturn. NASA’s Pioneer 11, and Voyagers 1 and 2 both zipped past the planet and its moons, snapping pictures as they went.
But Cassini was here to stay. To orbit around and around the planet, taking photos, measuring magnetic fields, and studying chemicals.
For Saturn itself, Cassini was able to make regular observations of the planet as it passed through entire seasons. This allowed it to watch how the weather and atmospheric patterns changed over time. The spacecraft watched lightning storms dance through the cloudtops at night.
Two highlights. In 2010, Cassini watched a huge storm erupt in the planet’s northern hemisphere. This storm dug deep into Saturn’s lower atmosphere, dredging up ice from a layer 160 kilometers below and mixing it onto the surface. This was the first time that astronomers were able to directly study this water ice on Saturn, which is normally in a layer hidden from view.
The second highlight, of course, is the massive hexagonal storm churning away in Saturn’s northern pole. This storm was originally seen by Voyager, but Cassini brought its infrared and visible wavelength instruments to bear.
Why a hexagon? That’s still a little unclear, but it seems like when you rotate fluids of different speeds, you get multi-sided structures like this.
Cassini showed how the hexagonal storm has changed in color as Saturn moved through its seasons.
This is one of my favorite images sent back by Cassini. It’s the polar vortex at the heart of the hexagon. Just look at those swirling clouds.
Now, images of Saturn itself are great and all, but there was so much else for Cassini to discover in the region.
Cassini studied Saturn’s rings in great detail, confirming that they’re made up of ice particles, ranging in size as small a piece of dust to as large as a mountain. But the rings themselves are actually quite thin. Just 10 meters thick in some places. Not 10 kilometers, not 10 million kilometers, 10 meters, 30 feet.
The spacecraft helped scientists uncover the source of Saturn’s E-ring, which is made up of fresh icy particles blasting out of its moon Enceladus. More on that in a second too.
Here’s another one of my favorite images of the mission. You’re looking at strange structures in Saturn’s B-ring. Towering pillars of ring material that rise 3.5 kilometers above the surrounding area and cast long shadows. What is going on here?
They’re waves, generated in the rings and enhanced by nearby moons. They move and change over time in ways we’ve never been able to study anywhere else in the Solar System.
Cassini has showed us that Saturn’s rings are a much more dynamic place than we ever thought. Some moons are creating rings, other moons are absorbing or distorting them. The rings generate bizarre spoke patterns larger than Earth that come and go because of electrostatic charges.
Speaking of moons, I’m getting to the best part. What did Cassini find at Saturn’s moons?
Let’s start with Titan, Saturn’s largest moon. Before Cassini, we only had a few low resolution images of this fascinating world. We knew Titan had a dense atmosphere, filled with nitrogen, but little else.
Cassini was carrying a special payload to assist with its exploration of Titan: the Huygens lander. This tiny probe detached from Cassini just before its arrival at Saturn, and parachuted through the cloudtops on January 14, 2005, analyzing all the way. Huygens returned images of its descent through the atmosphere, and even images of the freezing surface of Titan.
But Cassini’s own observations of Titan took the story even further. Instead of a cold, dead world, Cassini showed that it has active weather, as well as lakes, oceans and rivers of hydrocarbons. It has shifting dunes of pulverized rock hard water ice.
If there’s one place that needs exploring even further, it’s Titan. We should return with sailboats, submarines and rovers to better explore this amazing place.
We learned, without a shadow of a doubt, that Mimas absolutely looks like the Death Star. No question. But instead of a megalaser, this moon has a crater a third of its own size.
Cassini helped scientists understand why Saturn’s moon Iapetus has one light side and one dark side. The moon is tidally locked to Saturn, its dark side always leading the moon in orbit. It’s collecting debris from another Saturnian moon, Phoebe, like bugs hitting the windshield of a car.
Perhaps the most exciting discovery that Cassini made during its mission is the strange behavior of Saturn’s moon Enceladus. The spacecraft discovered that there are jets of water ice blasting out of the moon’s southern pole. An ocean of liquid water, heated up by tidal interactions with Saturn, is spewing out into space.
And as you know, wherever we find water on Earth, we find life. We thought that water in the icy outer Solar System would be hard to reach, but here it is, right at the surface, venting into space, and waiting for us to come back and investigate it further.
On September 15, 2017, the Cassini mission will end. How do we know it’s going to happen on this exact date? Because NASA is going to crash the spacecraft into Saturn, killing it dead.
That seems a little harsh, doesn’t it, especially for a spacecraft which has delivered so many amazing images to us over nearly two decades of space exploration? And as we’ve seen from NASA’s Opportunity rover, still going, 13 years longer than anticipated. Or the Voyagers, out in the depths of the void, helping us explore the boundary between the Solar System and interstellar space. These things are built to last.
The problem is that the Saturnian system contains some of the best environments for life in the Solar System. Saturn’s moon Enceladus, for example, has geysers of water blasting out into space.
Cassini spacecraft is covered in Earth-based bacteria and other microscopic organisms that hitched a ride to Saturn, and would be glad to take a nice hot Enceladian bath. All they need is liquid water and a few organic chemicals to get going, and Enceladus seems to have both.
NASA feels that it’s safer to end Cassini now, when they can still control it, than to wait until they lose communication or run out of propellant in the future. The chances that Cassini will actually crash into an icy moon and infect it with our Earth life are remote, but why take the risk?
For the last few months, Cassini has been taking a series of orbits to prepare itself for its final mission. Starting in April, it’ll actually cross inside the orbit of the rings, getting closer and closer to Saturn. And on September 15th, it’ll briefly become a meteor, flashing through the upper atmosphere of Saturn, gone forever.
Even in its final moments, Cassini is going to be sciencing as hard as it can. We’ll learn more about the density of consistency of the rings close to the planet. We’ll learn more about the planet’s upper atmosphere, storms and clouds with the closest possible photographs you can take.
And then it’ll all be over. The perfect finale to one of the most successful space missions in human history. A mission that revealed as many new mysteries about Saturn as it helped us answer. A mission that showed us not only a distant alien world, but our own planet in perspective in this vast Solar System. I can’t wait to go back.
How have the photos from Cassini impacted your love of astronomy? Let me know your thoughts in the comments.
Water-Trapped Worlds Possible Around Red Dwarf Stars?
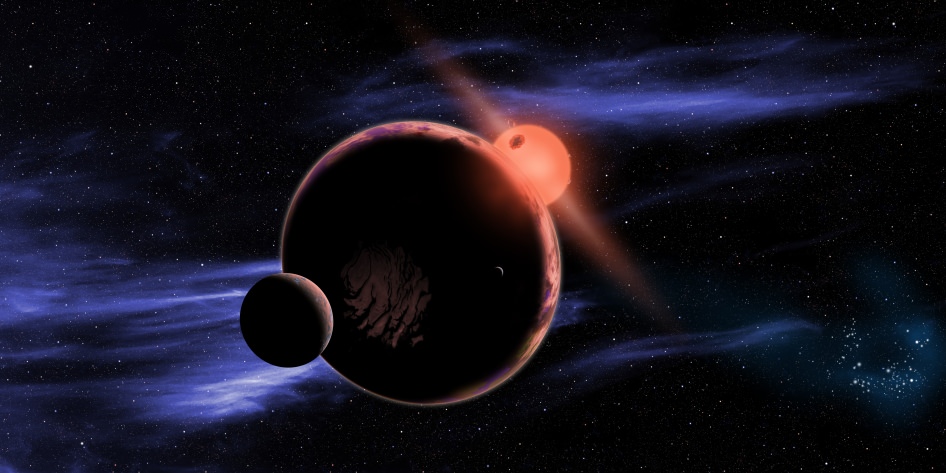
Hunters of alien life may have a new and unsuspected niche to scout out.
A recent paper submitted by Associate Professor of Astronomy at Columbia University Kristen Menou to the Astrophysical Journal suggests that tidally-locked planets in close orbits to M-class red dwarf stars may host a very unique hydrological cycle. And in some extreme cases, that cycle may cause a curious dichotomy, with ice collecting on the farside hemisphere of the world, leaving a parched sunward side. Life sprouting up in such conditions would be a challenge, experts say, but it is — enticingly — conceivable.
The possibility of life around red dwarf stars has tantalized researchers before. M-type dwarfs are only 0.075 to 0.6 times as massive as our Sun, and are much more common in the universe. The life span of these miserly stars can be measured in the trillions of years for the low end of the mass scale. For comparison, the Universe has only been around for 13.8 billion years. This is another plus in the game of giving biological life a chance to get underway. And while the habitable zone, or the “Goldilocks” region where water would remain liquid is closer in to a host star for a planet orbiting a red dwarf, it is also more extensive than what we inhabit in our own solar system.
But such a scenario isn’t without its drawbacks. Red dwarfs are turbulent stars, unleashing radiation storms that would render any nearby planets sterile for life as we know it.
But the model Professor Menou proposes paints a unique and compelling picture. While water on the permanent daytime side of a terrestrial-sized world tidally locked in orbit around an M-dwarf star would quickly evaporate, it would be transported by atmospheric convection and freeze out and accumulate on the permanent nighttime side. This ice would only slowly migrate back to the scorching daytime side and the process would continue.
Could these types of “water-locked worlds” be more common than our own?
The type of tidal locking referred to is the same as has occurred between the Earth and its Moon. The Moon keeps one face eternally turned towards the Earth, completing one revolution every 29.5 day synodic period. We also see this same phenomenon in the satellites for Jupiter and Saturn, and such behavior is most likely common in the realm of exoplanets closely orbiting their host stars.
The study used a dynamical model known as PlanetSimulator created at the University of Hamburg in Germany. The worlds modeled by the author suggest that planets with less than a quarter of the water present in the Earth’s oceans and subject to a similar insolation as Earth from its host star would eventually trap most of their water as ice on the planet’s night side.
Kepler data results suggest that planets in close orbits around M-dwarf stars may be relatively common. The author also notes that such an ice-trap on a water-deficient world orbiting an M-dwarf star would have a profound effect of the climate, dependent on the amount of volatiles available. This includes the possibility of impacts on the process of erosion, weathering, and CO2 cycling which are also crucial to life as we know it on Earth.
Thus far, there is yet to be a true “short list” of discovered exoplanets that may fit the bill. “Any planet in the habitable zone of an M-dwarf star is a potential water-trapped world, though probably not if we know the planet possesses a thick atmosphere.” Professor Menou told Universe Today. “But as more such planets are discovered, there should be many more potential candidates.”
Being that red dwarf stars are relatively common, could this ice-trap scenario be widespread as well?
“In short, yes,” Professor Menou said to Universe Today. “It also depends on the frequency of planets around such stars (indications suggest it is high) and on the total amount of water at the surface of the planet, which some formation models suggest should indeed be small, which would make this scenario more likely/relevant. It could, in principle, be the norm rather than the exception, although it remains to be seen.”
Of course, life under such conditions would face the unique challenges. The daytime side of the world would be subject to the tempestuous whims of its red dwarf host sun in the form of frequent radiation storms. The cold nighttime side would offer some respite from this, but finding a reliable source of energy on the permanently shrouded night side of such as world would be difficult, perhaps relying on chemosynthesis instead of solar-powered photosynthesis.
On Earth, life situated near “black smokers” or volcanic vents deep on the ocean floor where the Sun never shines do just that. One could also perhaps imagine life that finds a niche in the twilight regions of such a world, feeding on the detritus that circulates by.
Some of the closest red dwarf stars to our own solar system include Promixa Centauri, Barnard’s Star and Luyten’s Flare Star. Barnard’s star has been the target of searches for exoplanets for over a century due to its high proper motion, which have so far turned up naught.
The closest M-dwarf star with exoplanets discovered thus far is Gliese 674, at 14.8 light years distant. The current tally of extrasolar worlds as per the Extrasolar Planet Encyclopedia stands at 919.
This hunt will also provide a challenge for TESS, the Transiting Exoplanet Survey Satellite and the successor to Kepler due to launch in 2017.
Searching for and identifying ice-trapped worlds may prove to be a challenge. Such planets would exhibit a contrast in albedo, or brightness from one hemisphere to the other, but we would always see the ice-covered nighttime side in darkness. Still, exoplanet-hunting scientists have been able to tease out an amazing amount of information from the data available before- perhaps we’ll soon know if such planetary oases exist far inside the “snowline” orbiting around red dwarf stars.
Read the paper on Water-Trapped Worlds at the following link.