Thanks to Channel 37, radio astronomers keep tabs on everything from the Sun to pulsars to the lonely spaces between the stars. This particular frequency, squarely in the middle of the UHF TV broadcast band, has been reserved for radio astronomy since 1963, when astronomers successfully lobbied the FCC to keep it TV-free.
Back then UHF TV stations were few and far between. Now there are hundreds, and I’m sure a few would love to soak up that last sliver of spectrum. Sorry Charley, the moratorium is still in effect to this day. Not only that, but it’s observed in most countries across the world.
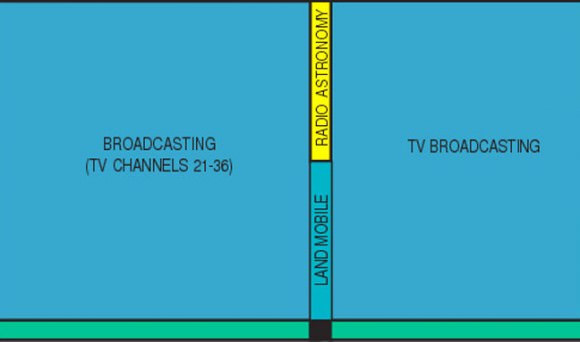
So what’s so important about Channel 37? Well, it’s smack in the middle of two other important bands already allocated to radio astronomy – 410 Megahertz (MHz) and 1.4 Gigahertz (Gz). Without it, radio astronomers would lose a key window in an otherwise continuous radio view of the sky. Imagine a 3-panel bay window with the middle pane painted black. Who wants THAT?
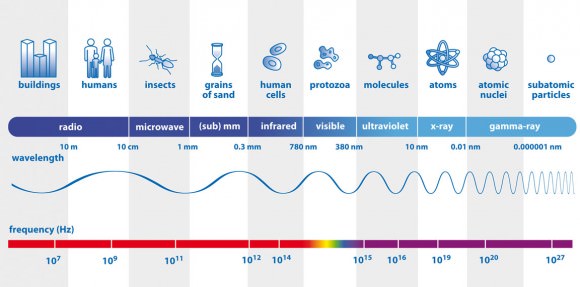
Channel 37 occupies a band spanning from 608-614 MHz. A word about Hertz. Radio waves are a form of light just like the colors we see in the rainbow or the X-rays doctors use to probe our bones. Only difference is, our eyes aren’t sensitive to them. But we can build instruments like X-ray machines and radio telescopes to “see” them for us.
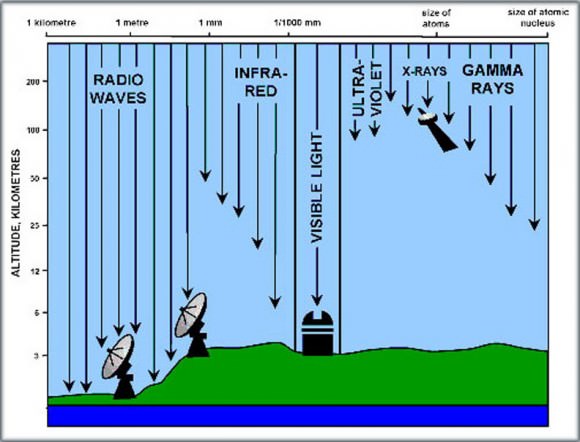
Every color of light has a characteristic wavelength and frequency. Wavelength is the distance between successive crests in a light wave which you can visualize as a wave moving across a pond. Waves of visible light range from one-millionth to one-billionth of a meter, comparable to the size of a virus or DNA molecule.
X-rays crests are jammed together even more tightly – one X-ray is only as big as an small atom. Radio waves fill out the opposite end of the spectrum with wavelengths ranging from baseball-sized to more than 600 miles (1000 km) long.
The frequency of a light wave is measured by how many crests pass a given point over a given time. If only one crest passes that point every second, the light beam has a frequency of 1 cycle per second or 1 Hertz. Blue light has a wavelength of 462 billionths of a meter and frequency of 645 trillion Hertz (645 Terahertz).
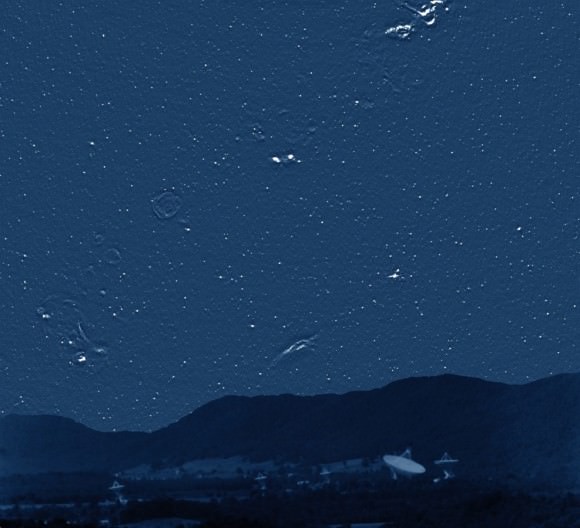
The higher the frequency, the greater the energy the light carries. X-rays have frequencies starting around 30 quadrillion Hertz (30 petahertz or 30 PHz), enough juice to damage body cells if you get too much exposure. Even ultraviolet light has power to burn skin as many of us who’ve spent time outdoors in summer without sunscreen are aware.
Radio waves are the gentle giants of the electromagnetic spectrum. Their enormous wavelengths mean low frequencies. Channel 37 radio waves have more modest frequencies of around 600 million Hertz (MHz), while the longest radio waves deliver crests almost twice the width of Lake Superior at a rate of 3 to 300 Hertz.
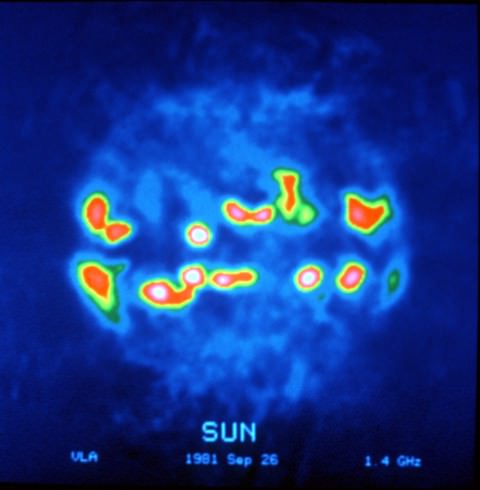
If Channel 37 were ever lost to TV, the gap would mean a loss of information about the distribution of cosmic rays in the Milky Way galaxy and rapidly rotating stars called pulsars created in the wake of supernovae. Closer to home, observations in the 608-614 MHz band allow astronomers track bursts of radio energy produced by particles blasted out by solar flares traveling through the sun’s outer atmosphere. Some of these can have powerful effects on Earth. No wonder astronomers want to keep this slice of the electromagnetic spectrum quiet. For more details on how useful this sliver is to radio astronomy, click HERE.
Just as optical astronomers seek the darkest sites for their telescopes to probe the most remote corners of the universe, so too does radio astronomy need slices of silence to listen to the faintest whispers of the cosmos.