
The most stunning panoramic vistas likely ever snapped by NASA’s Curiosity rover reveal spectacularly layered Martian rock formations in such exquisite detail that they look and feel just like America’s desert Southwest landscapes. They were just captured a week ago and look like a scene straight out of the hugely popular science fiction movie ‘The Martian’ – only they are real !!
Indeed several magnificent panoramas were taken by Curiosity in just the past week and you can see our newly stitched mosaic versions of several – above and below.
The rock formations lie in the “Murray Buttes” region of lower Mount Sharp where Curiosity has been exploring for roughly the past month. She just finished a campaign of detailed science observations and is set to bore a new sampling hole into the Red Planet, as you read this.
While scouting around the “Murray Buttes,” the SUV sized rover captured thousands of color and black and white raw images to document the geology of this thus far most unrivaled spot on the Red Planet ever visited by an emissary from Earth.
So the image processing team of Ken Kremer and Marco Di Lorenzo has begun stitching together wide angle mosaic views starting with images gathered by the high resolution mast mounted Mastcam right color camera, or M-100, on Sept, 8, 2016, or Sol 1454 of the robots operations on Mars.
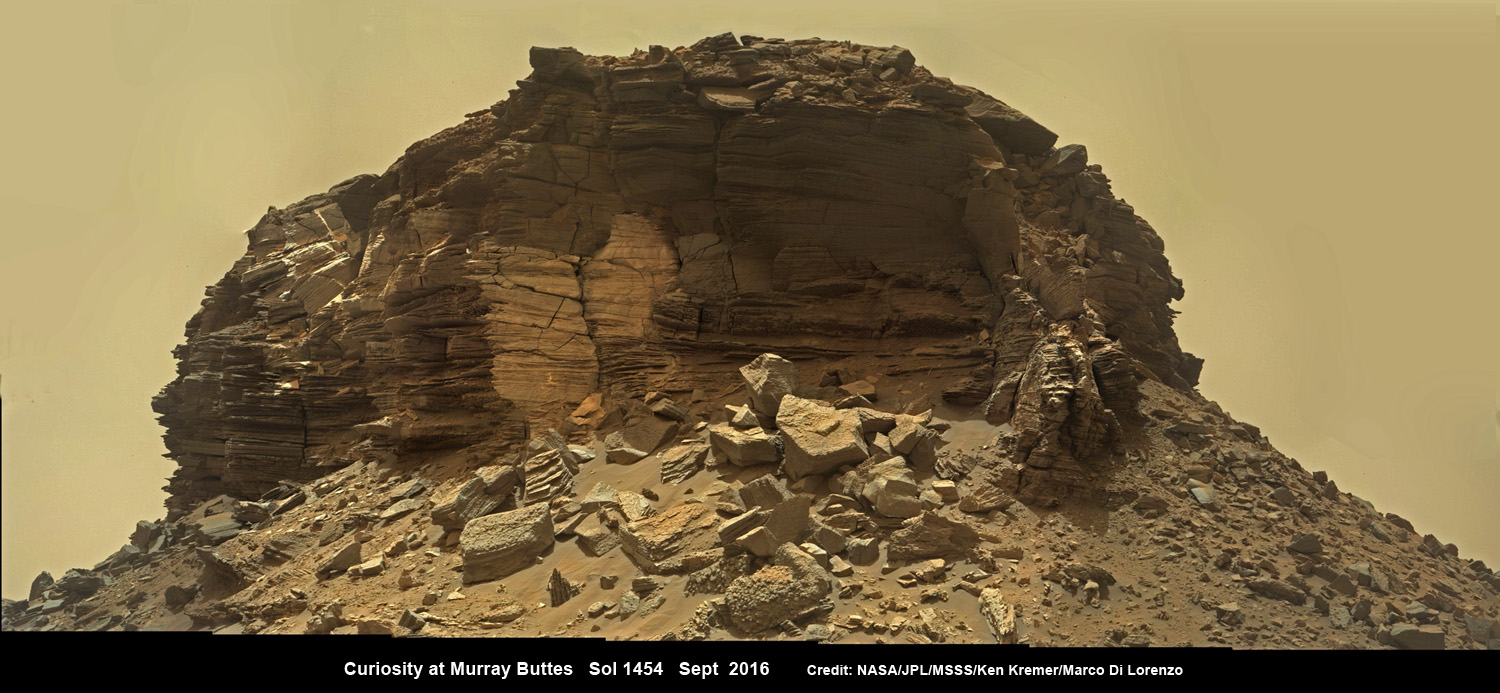
The mosaics give context and show us exactly what the incredible alien surroundings look like where the six wheeled rover is exploring today.
The imagery of the Murray Buttes and mesas show them to be eroded remnants of ancient sandstone that originated when winds deposited sand after lower Mount Sharp had formed.
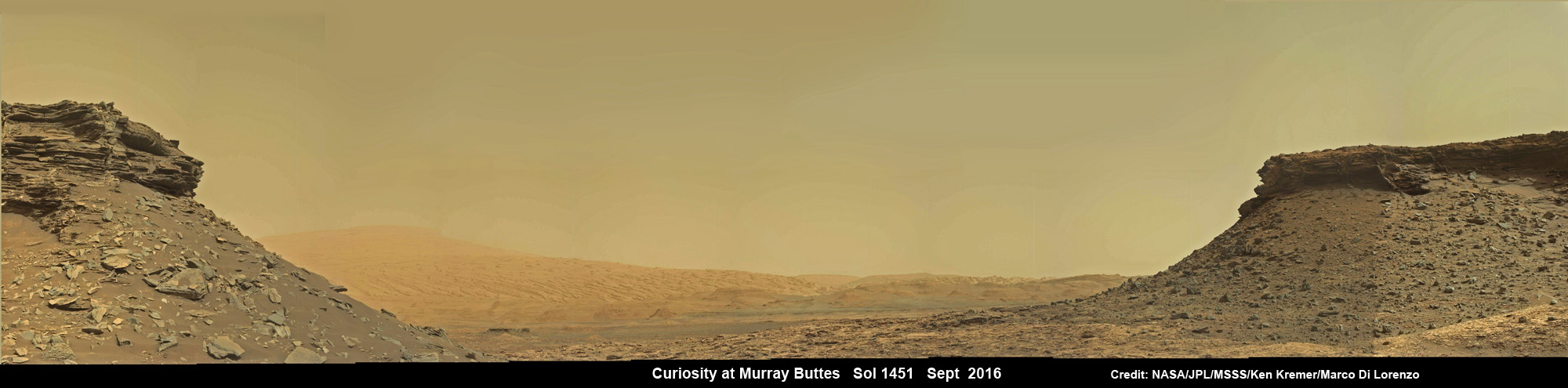
Scanning around the Murray Buttes mosaics one sees finely layered rocks, sloping hillsides, the distant rim of Gale Crater barely visible through the dusty haze, dramatic hillside outcrops with sandstone layers exhibiting cross-bedding. The presence of “cross-bedding” indicates that the sandstone was deposited by wind as migrating sand dunes, says the team.
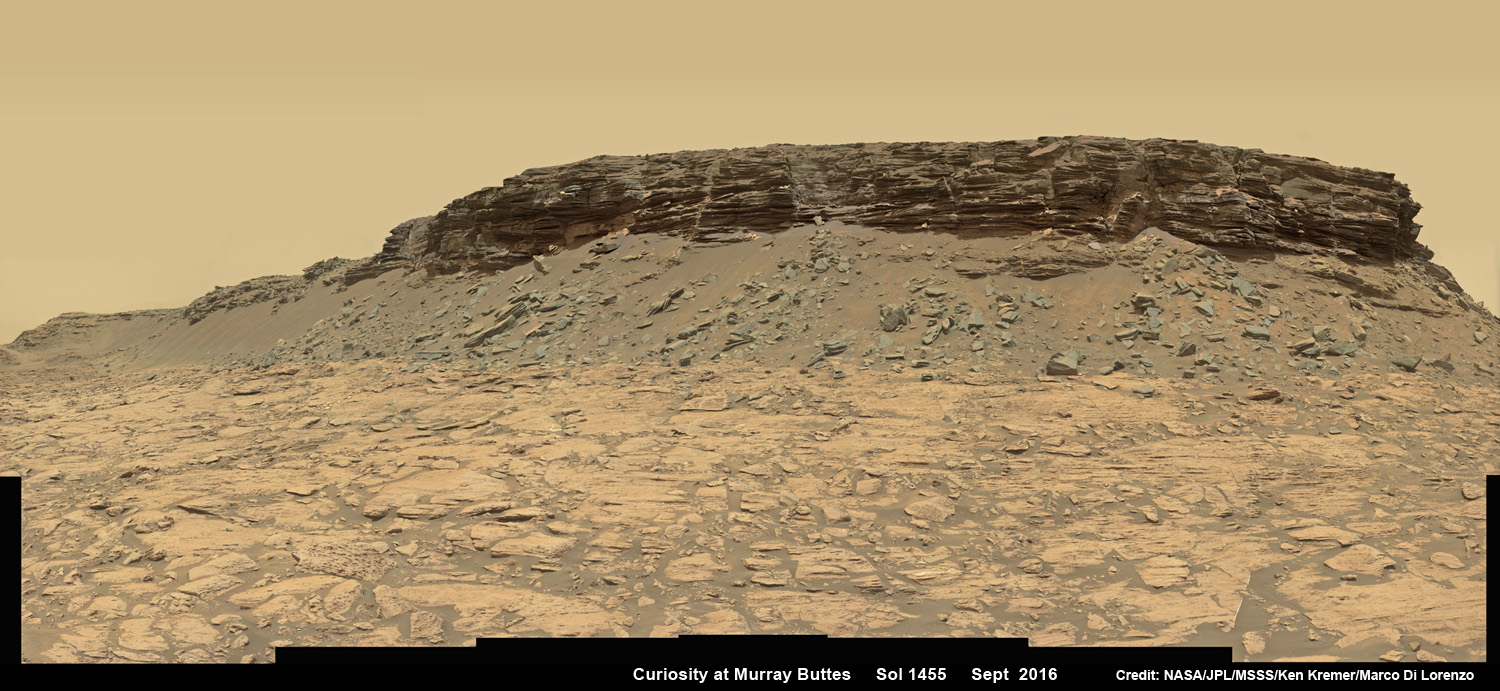
But there is no time to rest as she was commanded to head further south to the last of these Murray Buttes. And right now the team is implementing a plan for Curiosity to drill a new hole in Mars today – at a target named “Quela” at the base of the last of the buttes. The rover approached the butte from the south side a few days ago to get in place and plan for the drilling, take imagery to document stratigraphy and make compositional observations with the ChemCam laser instrument.
“It’s always an exciting day on Mars when you prepare to drill another sample – an engineering feat that we’ve become so accustomed to that I sometimes forget how impressive this really is!” wrote Lauren Edgar, in a mission update today. Edgar is a Research Geologist at the USGS Astrogeology Science Center and a member of the MSL science team.
Curiosity will then continue further south to begin exploring higher and higher sedimentary layers up Mount Sharp. The “Murray Buttes” are the entry way along Curiosity’s planned route up lower Mount Sharp.
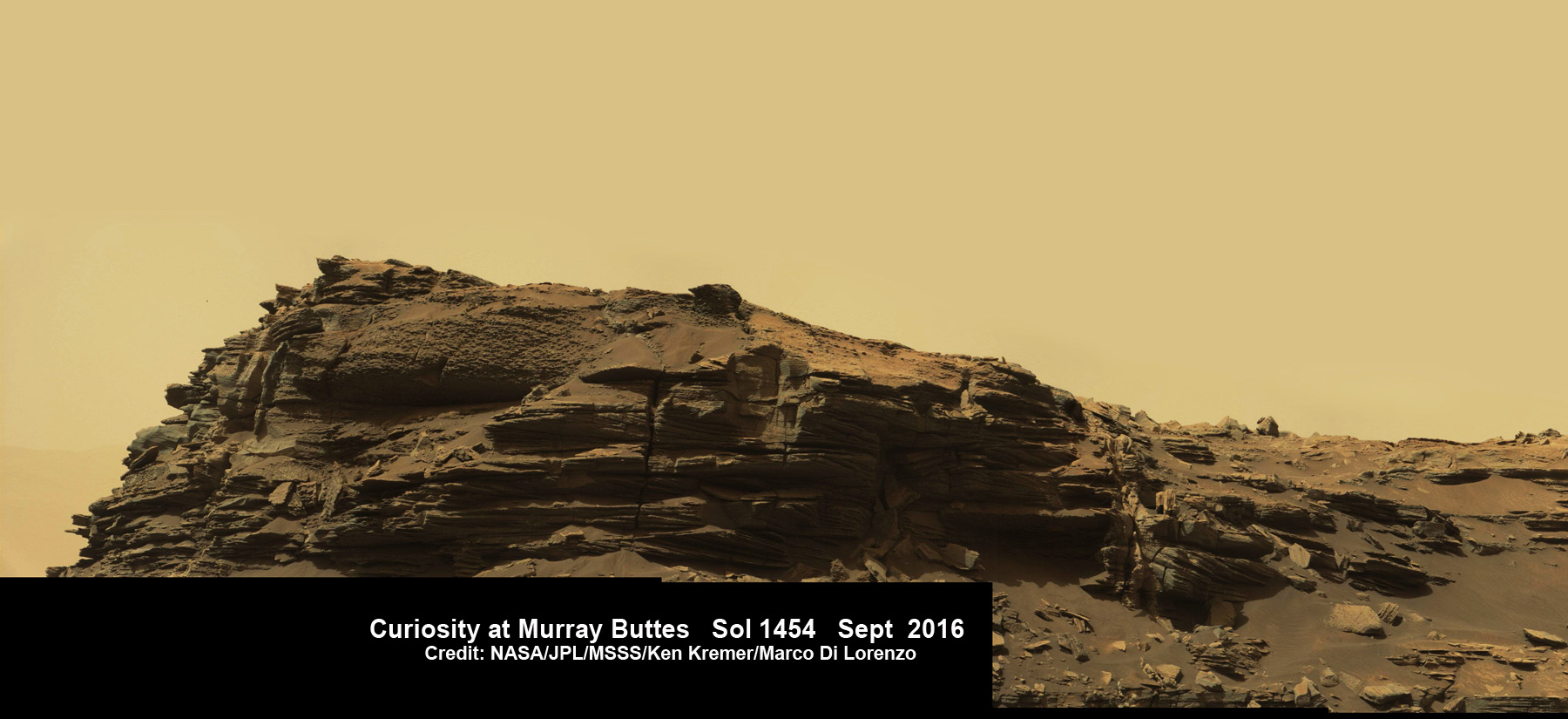
Meanwhile Curiosity is still conducting science observations of the last drill sample gathered from the “Marimba” target in August focusing on MAHLI and APXS examination of the dump pile leftovers from the sieved sample. She just completed chemical analysis of the sieved sample using the miniaturized SAM and CheMin internal chemistry laboratories.
It’s interesting to note that although the buttes are striking, their height also presents communications issues by blocking radio signals with NASA’s orbiting relay satellites. NASA’s Opportunity rover faced the same issues earlier this year while exploring inside the high walled Marathon Valley along Ecdeavour Crater.
“While the buttes are beautiful, they pose a challenge to communications, because they are partially occluding communications between the rover and the satellites we use to relay data (MRO and ODY), so sometimes the data volume that we can relay is pretty low” wrote Edgar.
“But it’s a small price to pay for the great stratigraphic exposures and gorgeous view!”
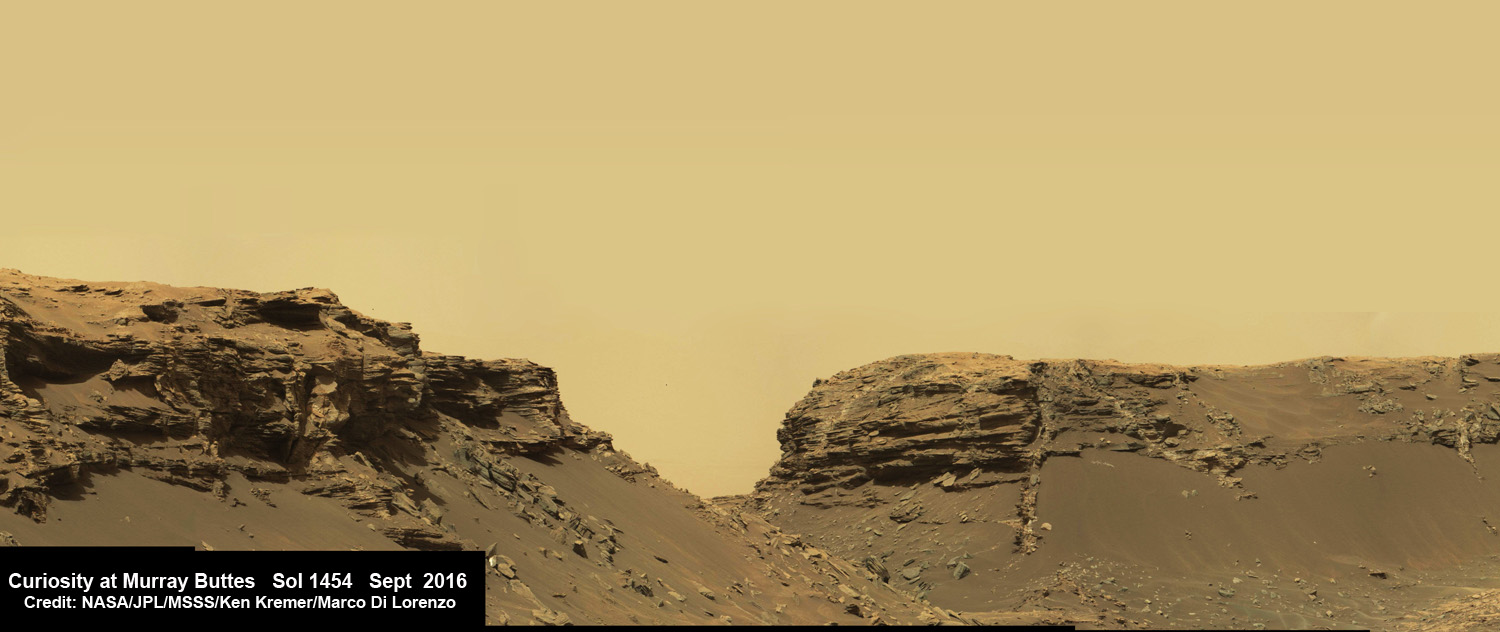
Ascending and diligently exploring the sedimentary lower layers of Mount Sharp, which towers 3.4 miles (5.5 kilometers) into the Martian sky, is the primary destination and goal of the rovers long term scientific expedition on the Red Planet.
Three years ago, the team informally named the Murray Buttes site to honor Caltech planetary scientist Bruce Murray (1931-2013), a former director of NASA’s Jet Propulsion Laboratory, Pasadena, California. JPL manages the Curiosity mission for NASA.
As of today, Sol 1461, September 15, 2016, Curiosity has driven over 7.9 miles (12.7 kilometers) since its August 2012 landing inside Gale Crater, and taken over 353,000 amazing images.
Stay tuned here for Ken’s continuing Earth and planetary science and human spaceflight news.
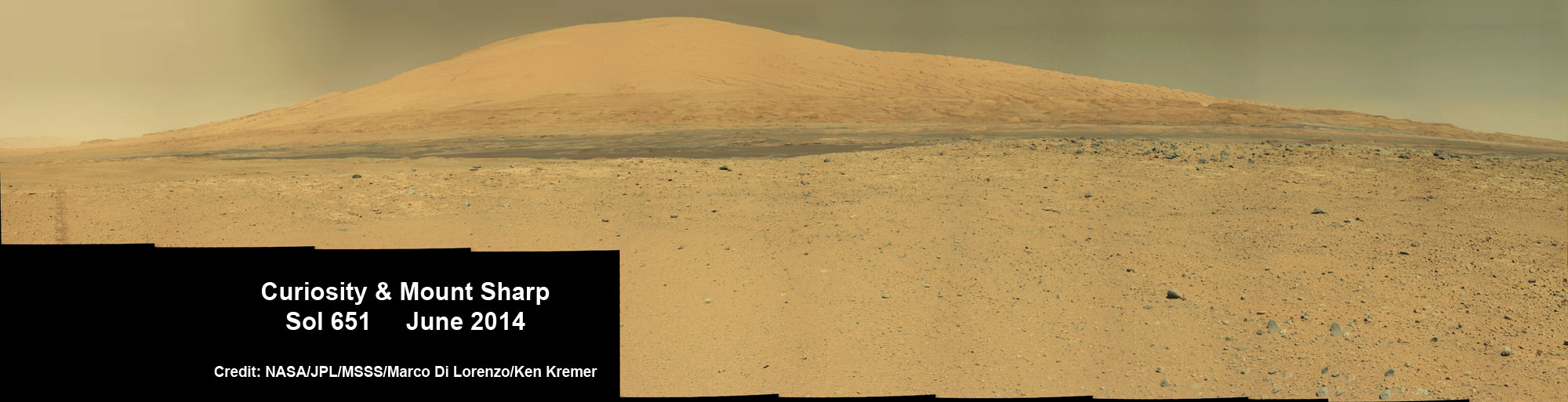