The giant outer planets haven’t always been in their current position. Uranus and Neptune for example are thought to have wandered through the outer Solar System to their current orbital position. On the way, they accumulated icy, comet-like objects. A new piece of research suggests as many as three kilomerer-sized objects crashed into them every hour increasing their mass. Not only would it increase the mass but it would enrich their atmospheres.
Continue reading “When Uranus and Neptune Migrated, Three Icy Objects Were Crashing Into Them Every Hour!”The Giant Planets in the Solar System Stunted the Growth of Mars
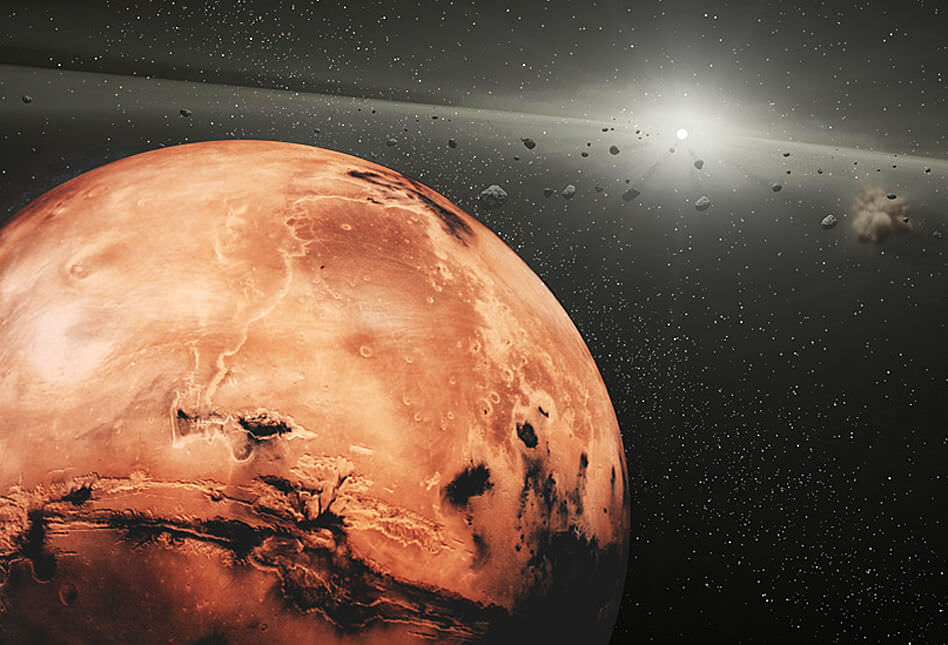
For centuries, astronomers and scientists have sought to understand how our Solar System came to be. Since that time, two theories have become commonly-accepted that explain how it formed and evolved over time. These are the Nebular Hypothesis and the Nice Model, respectively. Whereas the former contends that the Sun and planets formed from a large cloud of dust and gas, the latter maintains the giant planets have migrated since their formation.
This is what has led to the Solar System as we know it today. However, an enduring mystery about these theories is how Mars came to be the way it is. Why, for example, is it significantly smaller than Earth and inhospitable to life as we know it when all indications show that it should be comparable in size? According to a new study by an international team of scientists, the migration of the giant planets could have been what made the difference.
For over a decade, astronomers have been operating under the assumption that shortly after the formation of the Solar System, the gas and ice giants of the outer Solar System (Jupiter, Saturn, Uranus and Neptune) began to migrate outward. This is the substance of the Nice Model, which asserts that this migration had a profound effect on the evolution of the Solar System and the formation of the terrestrial planets.
This model – named for the location of the Observatoire de la Côte d’Azur (in Nice, France), where it was initially developed – began as an evolutionary model that helped explain the observed distributions of small objects like comets and asteroids. As Matt Clement, a graduate student in the HL Dodge Department of Physics and Astronomy at the University of Oklahoma and the lead author on the paper, explained to Universe Today via email:
“In the model, the giant planets (Jupiter, Saturn, Uranus and Neptune) originally formed much closer to the Sun. In order to reach their current orbital locations, the entire solar system undergoes a period of orbital instability. During this unstable period, the size and the shape of the giant planet’s orbits change rapidly.”
For the sake of their study, which was recently published in the scientific journal Icarus under the title “Mars Growth Stunted by an Early Giant Planet Instability“, the team expanded on the Nice Model. Through a series of dynamical simulations, they attempted to show how, during the early Solar System, the growth of Mars was halted thanks to the orbital instabilities of the giant planets.
The purpose of their study was also to address a flaw in the Nice Model, which is how the terrestrial planets could have survived a serious shake up of the Solar System. In the original version of the Nice Model, the instability of the giant planets occurred a few hundred million years after the planets formed, which coincided with the Late Heavy Bombardment – when the inner Solar System was bombarded by a disproportionately large number of asteroids.
This period is evidenced by spike in the Moon’s cratering record, which was inferred from an abundance of samples from the Apollo missions with similar geological dates. As Clement explained:
“A problem with this is that it is difficult for the terrestrial planets (Mercury, Venus, Earth and Mars) to survive the violent instability without being ejected out of the solar system or colliding with one another. Now that we have better, high resolution images of lunar craters and more accurate methods for dating the Apollo samples, the evidence for a spike in lunar cratering rates is diminishing. Our study investigated whether moving the instability earlier, while the inner terrestrial planets were still forming, could help them survive the instability, and also explain why Mars is so small relative to the Earth.”
Clement was joined by Nathan A. Kaib, a OU astrophysics professor, as well as Sean N. Raymond of the University of Bordeaux and Kevin J. Walsh from the Southwest Research Institute. Together, they used the computing resources of the OU Supercomputing Center for Education and Research (OSCER) and the Blue Waters supercomputing project to perform 800 dynamical simulations of the Nice model to determine how it would impact Mars.
These simulations incorporated recent geological evidence from Mars and Earth that indicate that Mars’ formation period was about 1/10th that of Earth’s. This has led to the theory that Mars was left behind as a “stranded planetary embryo” during the formation of the Sun’s inner planets. As Prof. Kaib explained to Universe Today via email, this study was therefore intended to test how Mars emerged from planetary formation as a planetary embryo:
“We simulated the “giant impact phase” of terrestrial planet formation (the final stage of the formation process). At the beginning of this phase, the inner Solar System (0.5-4 AU) consists of a disk of about 100 moon-to-mars-sized planetary embryos embedded in a sea of much smaller, more numerous rocky planetesimals. Over the course of 100-200 million years the bodies making up this system collide and merge into a handful (typically 2-5) rocky planetary mass bodies. Normally, these types of simple initial conditions build planets on Mars-like orbits that are about 10x more massive than Mars. However, when the terrestrial planet formation process is interrupted by the Nice model instability, many of the planet building blocks near the Mars region are lost or tossed into the Sun. This limits the growth of Mars-like planets and produces a closer match to our actual inner solar system.”
What they found was that this revised timeline explained the disparity between Mars and Earth. In short, Mars and Earth vary considerably in size, mass and density because the giant planets became unstable very early in the Solar System’s history. In the end, this is what allowed Earth to become the only life-bearing terrestrial planet in the Solar System, and for Mars to become the cold, desiccated and thinly-atmosphered place that it is today.
As Prof. Kaib explained, this is not the only model for explaining the disparity between Earth and Mars, but the evidence all fits:
“Without this instability, Mars likely would have had a mass closer to Earth’s and would be a very different, perhaps more Earth-like, planet compared to what it is today,” he said. “I should also say that this is not the only mechanism capable of explaining the low mass of Mars. However, we already know that the Nice model does an excellent job of reproducing many features of the outer Solar System, and if it occurs at the right time in the Solar System’s history it also ends up explaining our inner Solar System.”
This study could also have drastic implications when it comes to the study of extra-solar systems. At present, our models for how planets form and evolve are based on what we have been able to learn from our own Solar System. Hence, by learning more about how gas giants and terrestrial planets grew and assumed their current orbits, scientists will be able to create more comprehensive models of how life-bearing planets could merge around other stars.
It certainly would help narrow the search for “Earth-like” planets and (dare we dream?) planets that support life.
Further Reading: University of Oklahoma, Icarus
Beautiful Planetary Rings Are Dead Dwarf Planets! Dead Dwarf Planets!!!
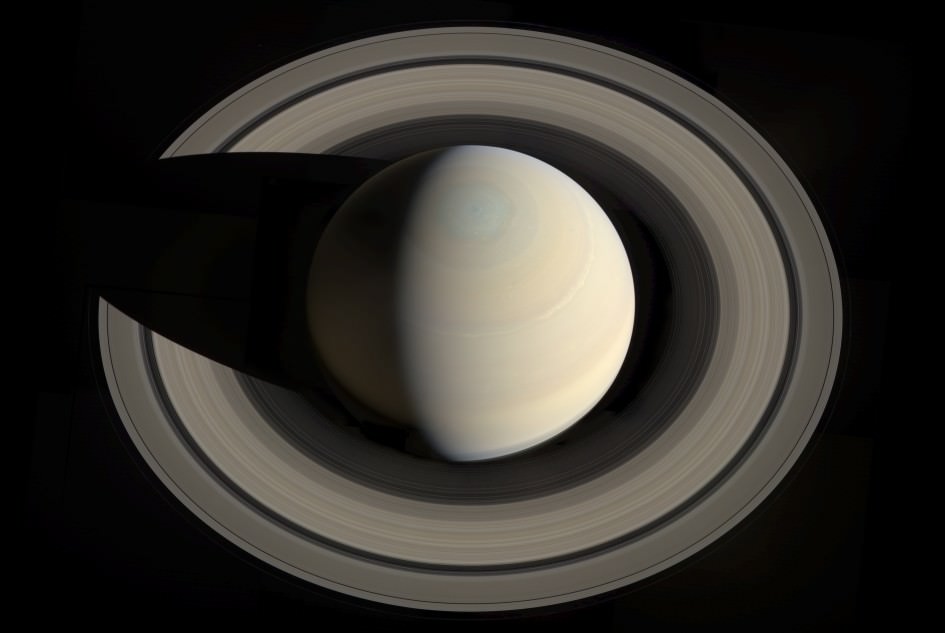
In 1655, astronomer Christiaan Huygens became the first person to observe the beautiful ring system that surrounds Saturn. And while they are certainly the most spectacular, astronomers have since discovered that all the gas and ice giants of the Solar System (i.e. Jupiter, Saturn, Uranus and Neptune) have their own system of rings.
These systems have remained a source of fascination for astronomers, largely because their origins are still something of a mystery. But thanks to a recent study by researchers from the Tokyo Institute of Technology and Kobe University, the origins of these rings may be solved. According to their study, the rings are pieces of Dwarf Planets that got torn off in passing, which were then ripped to pieces!
This research could help to resolve many of the burning questions about the ring systems around our system’s giant planets, as well as details about the Solar Systems past. For the sake of their study – titled “Ring Formation around Giant Planets by Tidal Disruption of a Single Passing Large Kuiper Belt Object” – the Japanese team of researchers considered a number of factors.
First, they considered the diversity of the various ring systems in our Solar System. For instance, Saturn’s rings are massive (about 100,000 trillion kg!) and composed overwhelmingly (90-95%) of water ice. In contrast, the much less massive rings of Uranus and Neptune are composed of darker material, and are believed to have higher percentages of rocky material in them.
To shed some light on this, the team looked to the Nice Model – a theory of Solar System formation that states that the gas giant migrated to their present location during the Late Heavy Bombardment. This period took place between 4 and 3.8 billion years ago, and was characterized by a disproportionately high number of asteroids from Trans-Neptunian space striking planets in the Inner Solar System.
They then considered other recent models of Solar System formation which postulate that the giant planets experienced close encounters with Pluto-sized objects during this time. From this, they developed the theory that the rings could be the result of some of these objects getting trapped and ripped apart by the gas giants’ gravity. To test this theory, they performed a number of computer simulations to see what would happen in these instances.
As Ryuki Hyodo – a researcher at the Department of Planetology, Kobe University, and the lead author on the paper – told Universe Today via email:
“We performed two simulations. First, using SPH (Smoothed-particle hydrodynamics) simulations, we investigated tidal disruption of Pluto-sized objects during the close encounters with giant planets and calculated the amount of fragments that are captured around giant planets. We found enough mass/fragments to explain current rings is captured. Then, we performed the longer-term evolution of the captured mass/fragments by using N-body simulations. We found that the captured fragments can collide each other with destruction and form thin equatorial circular rings around giant planets.”
The results of these simulation were consistent with the mass of the ring systems observed around Saturn and Uranus. This included the inner regular satellites of both planets – which would have also been the product of the past encounters with KBOs. It also accounted for the differences in the rings’ composition, showing how the planet’s Roche limits can influence what kind of material can be effectively captured.
This study is especially significant because it offers verifiable evidence for one of the enduring mysteries of our Solar System. And as Hyodo points out, it could come in mighty handy when it comes time to examine extra-solar planetary systems as well.
“Our theory suggested that, in the past, we had two possible epochs to form rings,” he said. “One is during the planet accretion phase and the other is during the Late heavy bombardment. Also, our model is naturally applicable to other planetary systems. So, our theory predicts that exoplanets also have massive rings around them.”
In the meantime, some might find the idea that ring systems are the corpses of Dwarf Planets troublesome. But I think we can all agree, a Soylent Green allusion might be just a bit over the top!
Further Reading: arXiv
Some Planet-like Kuiper Belt Objects Don’t Play “Nice”
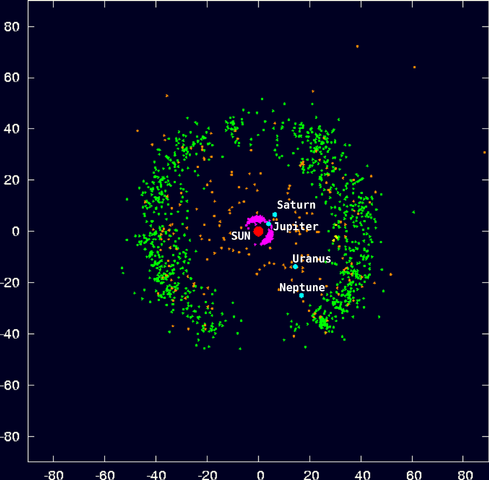
The Kuiper belt — the region beyond the orbit of Neptune inhabited by a number of small bodies of rock and ice — hides many clues about the early days of the Solar System. According to the standard picture of Solar System formation, many planetesimals were born in the chaotic region where the giant planets now reside. Some were thrown out beyond the orbit of Neptune, while others stayed put in the form of Trojan asteroids (which orbit in the same trajectory as Jupiter and other planets). This is called the Nice model.
However, not all Kuiper belt objects (KBOs) play nicely with the Nice model.
(I should point out that the model is named named for the city in France and therefore pronounced “neese”.) A new study of large scale surveys of KBOs revealed that those with nearly circular orbits lying roughly in the same plane as the orbits of the major planets don’t fit the Nice model, while those with irregular orbits do. It’s a puzzling anomaly, one with no immediate resolution, but it hints that we need to refine our Solar System formation models.
This new study is described in a recently released paper by Wesley Fraser, Mike Brown, Alessandro Morbidelli, Alex Parker, and Konstantin Baygin (to be published in the Astrophysical Journal, available online). These researchers combined data from seven different surveys of KBOs to determine roughly how many of each size of object are in the Solar System, which in turn is a good gauge of the environment in which they formed.
The difference between this and previous studies is the use of absolute magnitudes — a measure of how bright an object really is — as opposed to their apparent magnitudes, which are simply how bright an object appears. The two types of magnitude are related by the distance an object is from Earth, so the observational challenge comes down to accurate distance measurements. Absolute magnitude is also related to the size of an KBO and its albedo (how much light it reflects), both important physical quantities for understanding formation and composition.
Finding the absolute magnitudes for KBOs is more challenging than apparent magnitudes for obvious reasons: these are small objects, often not resolved as anything other than points of light in a telescope. That means requires measuring the distance to each KBO as accurately as possible. As the authors of the study point out, even small errors in distance measurements can have a large effect on the estimated absolute magnitude.
In terms of orbits, KBOs fall into two categories: “hot” and “cold”, confusing terms having nothing to do with temperature. The “cold” KBOs are those with nearly circular orbits (low eccentricity, in mathematical terms) and low inclinations, meaning their trajectories lie nearly in the ecliptic plane, where the eight canonical planets also orbit. In other words, these objects have nearly planet-like orbits. The “hot” KBOs have elongated orbits and higher inclinations, behavior more akin to comets.
The authors of the new study found that the hot KBOs have the same distribution of sizes as the Trojan asteroids, meaning there are the same relative number of small, medium, and large KBOs and similarly sized Trojans. That hints at a probable common origin in the early days of the Solar System. This is in line with the Nice model, which predicts that, as they migrated into their current orbits, the giant planets kicked many planetesimals out beyond Neptune.
However, the cold KBOs don’t match that pattern at all: there are fewer large KBOs relative to smaller objects. To make matters more strange, both hot and cold seem to follow the same pattern for the smaller bodies, only deviating at larger masses, which is at odds with expectations if the cold KBOs formed where they orbit today.
To put it another way, the Nice model as it stands could explain the hot KBOs and Trojans, but not the cold. That doesn’t mean all is lost, of course. The Nice model seems to do very well except for a few nagging problems, so it’s unlikely that it’s completely wrong. As we’ve learned from studying exoplanet systems, planet formation models are a work in progress — and astronomers are an ingenious lot.
Neptune Acquitted on One Count of Harassment

[/caption]
A very popular explanation for the dynamical evolution of our solar system is being challenged by a new model that takes the blame away from Neptune for knocking a collection of planetoids known as the Cold Classical Kuiper Belt out to their current, distant home. PhD student Alex Parker from the University of Victoria in British Columbia, Canada presented evidence showing that the large population of binary objects in the Kuiper Belt gives witness to a different series of events than the Nice Model – which says Neptune’s migrations were responsible for a sending KBO’s into chaotic orbits. “Kuiper binaries paint a different picture,” Parker said during a press briefing at the American Astronomical Society’s Division of Planetary Sciences meeting this week. “I should title my talk as ‘Neptune not guilty of harassment’ or perhaps more accurately, “Planet Neptune acquitted of one count of harassment.’”
The Nice Model holds that the objects in the scattered Kuiper Belt were placed in their current positions by interactions with Neptune’s migrating resonances. Originally, the Model says, the Kuiper belt was much denser and closer to the Sun, with an outer edge at approximately 30 AU. Its inner edge would have been just beyond the orbits of Uranus and Neptune, which were in turn far closer to the Sun when they formed. As Neptune migrated outward, it approached the objects in the proto-Kuiper belt, capturing some of them into resonances and sending others into chaotic orbits.
But the survey of the Kuiper Belt being done by Parker and his thesis supervisor Dr. J. J Kavelaars (Herzberg Institute of Astrophysics), which has been running for a decade, tells a different story. “Thirty per cent of Kuiper Belt Objects are binaries, some in very wide orbits around each other in a slow waltz, weakly bound to their partners,” Parker said. “These binaries should have been destroyed if the Kuiper Belt Objects were thrown out of solar system.”
Since binaries are extremely common in the Kuiper Belt, they are useful tools for astronomers, said Parker. “Pluto and Charon are the most famous of these binaries and since their orbits can be affected by their environment, we can use them to test what the interplanetary environment is like and what it was like in the past.”
Using computer simulations, the researchers determined that many binary systems in part of the Belt would have been destroyed by the manhandling they would have experienced if Neptune did indeed move the Kuiper Belt to its current location.
The survey characterizes the orbits of these binaries and found that many are extremely wide – the widest one is about 100,000 km – and they are very delicate. “Because they are so weakly bound they can be upset by collisions from small objects peppering the KBOs,” said Parker, “and they would not be there today if the members of this part of the Kuiper Belt were ever hassled by Neptune in the past.”
Additionally, the current environment of the Kuiper Belt does not lend itself to the creation of these binaries, so they have been interacting with each other for a very long time. The research done by Parker and his colleagues suggest that the Kuiper Belt formed near its present location and has remained undisturbed over the age of the solar system.
The new model also solves the missing mass problem for the Kuiper Belt, Parker said. “The Nice Model – as well as all the other models of the formation of the Kuiper Belt — suggests its density was much higher so the binaries could be generated, but we don’t see that density today.”
The Cold Classical Kuiper Belt lies in a very flat ring between 6 and 7 billion kilometers from the Sun, and contains thousands of bodies larger than 100 kilometers across. The Kuiper Belt is of special interest to astrophysicists because it is a fossil remnant of the primordial debris that formed the planets, said Parker. “Understanding the structure and history of the Kuiper Belt helps us better understand how the planets in our solar system formed, and how planets around other stars may be forming today.”
Read the team’s paper: “Destruction of Binary Minor Planets During Neptune Scattering,” Alex H. Parker, JJ Kavelaars
Sources: DPS meeting press briefing, DPS abstract, University of Victoria press release.