Waves move through a medium, like water or air. So it seemed logical to search for a medium that light waves move through. The Michelson-Morley Experiment attempted to search for this medium, known as the “luminiferous aether”. The experiment gave a negative result, and helped set the stage for the theory of General Relativity.
Continue reading “Astronomy Cast Ep. 368: Searching for the Aether Wind: the Michelson–Morley Experiment”
Where’s All The Antimatter?
One of the biggest mysteries in the Universe is the fact there there’s matter, and not antimatter. Where did it all go?
When we look around, everything we can see is made of matter. For every type of matter from electrons, protons and quarks there is a similar type of matter known as antimatter. So why aren’t there piles of antimatter rocks, cars and chocolate bars just lying around? Why does Scotty always have a little extra kicking around in his liquor cabinet? And where do I get mine?
The primary difference between matter and antimatter is that they have opposite electric charge. Which seems pretty mundane. The negatively charged electron has an antiparticle known as the positron, which has a positive electric charge.
Anti-protons have a negative charge, and are just flat out grumpy. We’ve been able to create these particles in the lab, and have even been able to create small amounts of anti-hydrogen consisting of a positron bound to an antiproton, when examined closely there’s were shown to have a goatee and a little colorful sash or dagger.
When we create particles in accelerators such as the Large Hadron Collider, we seem to get equal amounts of matter and antimatter. This suggests that when particles were formed soon after the big bang, there should have been equal amounts of matter and antimatter.
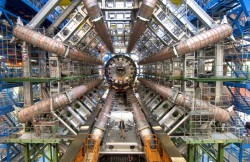
But the universe we observe is only made of matter, and… here’s the best part… we have no idea why. Why didn’t the matter and antimatter completely annihilate each other? How come we ended up with a little more matter? This delightful mystery is known as baryon asymmetry.
We do have a few ideas, and by we, I mean some giant brained crackerjacks have come up with a few plausible options. The most popular is that somehow antimatter behaves a little differently than matter. This could cause an imbalance between matter and antimatter. After particles collided in the early universe, there would still be matter left over, hence the matter we observe.
Another idea is that the observable universe just happens to be in a region dominated by matter. Other parts of the multiverse could have observable universes dominated by antimatter. Baryon asymmetry is one of the big mysteries of modern cosmology.
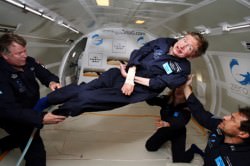
There is an even crazier idea. Antimatter might have anti-gravity. In other words, an atom of anti-hydrogen would fall up instead of down. If that is the case, then matter and antimatter would repel each other, and you might have matter universes and antimatter universes that are forever separate.There have been some initial experiments to test this idea, but so far there have been no conclusive results.
What do you think? Where’s all our antimatter and how do we track it down? I’d sure love to bring some home and show my friends…
And if you like what you see, come check out our Patreon page and find out how you can get these videos early while helping us bring you more great content!
NASA’s Particle-Hunting ISS-CREAM Will Be Anything But Vanilla
Balloon-based research on cosmic particles that began over a century ago will get a big boost next year — all the way up to low-Earth orbit, when NASA’s Cosmic Ray Energetics and Mass (CREAM) will be sent to the Space Station thus becoming (are you ready for this?) ISS-CREAM, specifically designed to detect super-high-energy cosmic rays and help scientists determine what their mysterious source(s) may be.
“The answer is one the world’s been waiting on for 100 years,” said program scientist Vernon Jones.
Read more about this “cool” experiment below:
Cosmic Ray Energetics and Mass (CREAM) will be the first cosmic ray instrument designed to detect at such higher energy ranges, and over such an extended duration in space. Scientists hope to discover whether cosmic rays are accelerated by a single cause, which is believed to be supernovae. The new research also could determine why there are fewer cosmic rays detected at very high energies than are theorized to exist.
“Cosmic rays are energetic particles from outer space,” said Eun-Suk Seo, principal investigator for the CREAM study. “They provide a direct sample of matter from outside the solar system. Measurements have shown that these particles can have energies as high as 100,000 trillion electron volts. This is an enormous energy, far beyond and above any energy that can be generated with manmade accelerators, even the Large Hadron Collider at CERN.”
Researchers also plan to study the decline in cosmic ray detection, called the spectral “knee” that occurs at about a thousand trillion electron-volts (eV), which is about 2 billion times more powerful than the emissions in a medical nuclear imaging scan. Whatever causes cosmic rays, or filters them as they move through the galaxy, takes a bite out of the population from 1,000 trillion electron-volts upwards. Further, the spectrum for cosmic rays extends much farther beyond what supernovas are believed to be able to produce.
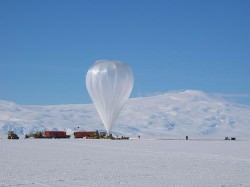
To tackle these questions, NASA plans to place CREAM aboard the space station, becoming ISS-CREAM. The instrument has flown six times for a total of 161 days on long-duration balloons circling the South Pole, where Earth’s magnetic field lines are essentially vertical.
The idea of energetic particles coming from space was unknown in 1911 when Victor Hess, the 1936 Nobel laureate in physics credited for the discovery of cosmic rays, took to the air to tackle the mystery of why materials became more electrified with altitude, an effect called ionization. The expectation was that the ionization would weaken as one got farther from Earth. Hess developed sensitive instruments and took them as high as 3.3 miles (5.3 kilometers) and he established that ionization increased up to fourfold with altitude, day or night.
A better understanding of cosmic rays will help scientists finish the work started when Hess unexpectedly turned an earthly question into a stellar riddle. Answering that riddle will help us understand a hidden, fundamental facet of how our galaxy, and perhaps the universe, is built and works.
The phenomenon soon gained a popular but confusing name, cosmic rays, from a mistaken theory that they were X-rays or gamma rays, which are electromagnetic radiation, like light. Instead, cosmic rays are high-speed, high-energy particles of matter.
As particles, cosmic rays cannot be focused like light in a telescope. Instead, researchers detect cosmic rays by the light and electrical charges produced when the particles slam into matter. The scientists then use detective work to identify the original particle by direct measurement of its electric charge and its energy determination from the avalanche of debris particles creating their own overlapping trails.
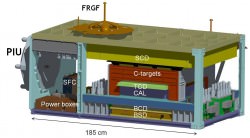
CREAM does this trace work using an ionization calorimeter designed to make cosmic rays shed their energies. Layers of carbon, tungsten and other materials present well-known nuclear “cross sections” within the stack. Electrical and optical detectors measure the intensity of events as cosmic particles, from hydrogen to iron, crash through the instrument.
Even though CREAM balloon flights reached high altitudes, enough atmosphere remained above to interfere with measurements. The plan to mount the instrument to the exterior of the space station will place it above the obscuring effects of the atmosphere, at an altitude of 250 miles (400 kilometers).
“On what can we now place our hopes of solving the many riddles which still exist as to the origin and composition of cosmic rays?”
– Victor F. Hess, Nobel Lecture, Dec. 1936
Source: NASA
Watch a Million Particles Collide
What happens when you give 1,000,000 particles their own gravity and spring repulsion and send them out to play? Watch the video above and find out.
This was created by David Moore, a self-taught computer programmer, aspiring physicist and student at San Diego Miramar College. It’s a custom code made with SDL/C++ and 8 days of render time. According to David there’s a bug at the end “where particles can get arbitrarily high energy… but before that it’s very physically accurate!”
It’s fascinating to watch the attraction process take place — one might envision a similar process occurring in the early Universe with the formation of the first galaxies and galactic clusters out of a hot, uniform state. Plus it’s great to see young talented minds like David’s working on such projects for fun!
There just might be hope for us after all.
Video by David Moore
Saturn’s Fluctuating F Ring
Bright clumps of material spotted within Saturn’s ropy F ring (NASA/JPL/SSI)
Released today, this image acquired by NASA’s Cassini spacecraft shows some interesting structures forming within Saturn’s thinnest but most dynamic ring.
Of Saturn’s countless ring structures the F ring may very well be the most dynamic, if not the most fascinating. Orbiting Saturn just outside the edge of the A ring at a distance of 140,000 km (87,000 miles), the F ring is a hazy, ropy band of fine ice particles that shift, twist and occasionally gather into bright clumps… only to drift apart once more.
The F ring can range in width from 30 to 500 km (20-500 miles), depending on what’s going on in and outside of it.
The image above, originally captured by Cassini on June 28 and released today by the Cassini Imaging Central Laboratory for Operations (CICLOPS), shows a particularly bright clump of material at the outer edge of the F ring, as well as some finer structures and streamers forming within the inner bands. Due to the lighting geometry its thought that the clumps are mostly composed of dusty material.
Detail of the ghostly F ring structures (NASA/JPL/SSI)
The features seen here are likely due to the ring’s interactions with passing shepherd moons — such as the 148-km-wide Prometheus — or with small moonlets embedded within the ring itself. Mostly made of fine particles of dust and ice smaller than those found in smoke, the material orbiting within the F ring is extremely susceptible to external gravitational influences.
Original image scale is 4 km (3 miles) per pixel.
See more images from the entire Cassini mission on the CICLOPS site here (and for a look at more interesting ring dynamics check out these recent Cassini images of my personal favorite moon, Daphnis.)
A Rare Type of Solar Storm Spotted by Satellite
[/caption]
When a moderate-sized M-class flare erupted from the Sun on May 17, it sent out a barrage of high-energy solar particles that belied its initial intensity. These particles traveled at nearly the speed of light, crossing the 93 million miles between the Sun and Earth in a mere 20 minutes and impacting our atmosphere, causing cascades of neutrons to reach the ground — a rare event known as a ground level enhancement, or GLE.
The first such event since 2006, the GLE was recorded by a joint Russian/Italian spacecraft called PAMELA and is an indicator that the peak of solar maximum is on the way.
The PAMELA spacecraft — which stands for Payload for Antimatter-Matter Exploration and Light-nuclei Astrophysics — is designed to detect high-energy cosmic rays streaming in from intergalactic space. But on May 17, scientists from NASA’s Goddard Space Flight Center convinced the Russian team in charge of PAMELA to grab data from the solar event occurring much closer to home.
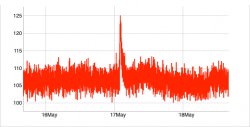
The result: the first observations from space of the solar particles that trigger the neutron storms that make up a GLE. Scientists hope to use the data to learn more about how GLEs are created, and why the May 17 “moderate” solar flare ended up making one.
“Usually we would expect this kind of ground level enhancement from a giant coronal mass ejection or a big X-class flare,” said Georgia de Nolfo, a space scientist at NASA’s Goddard Space Flight Center. “So not only are we really excited that we were able to observe these particularly high energy particles from space, but we also have a scientific puzzle to solve.”
Fewer than 100 GLEs have been recorded in the last 70 years, with the most powerful having occurred on February 23, 1956. Like most energetic solar outbursts, GLEs can have disruptive effects on sensitive electronics in orbit as well as on the ground, and based on recent studies may even have adverse effects on cellular systems and development.
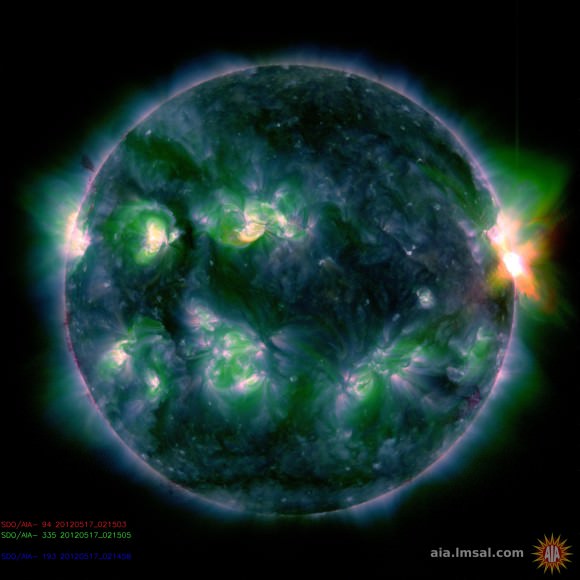
Read more on the NASA news release here.
Finding Out What Dark Matter Is – And Isn’t
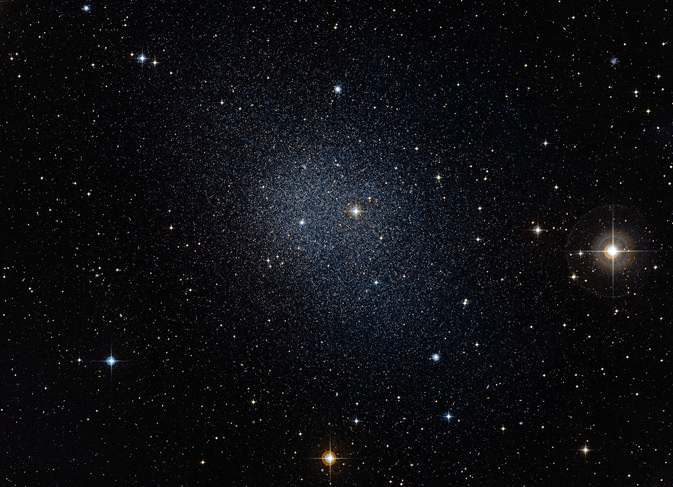
Astronomers using NASA’s Fermi Gamma-Ray Space Telescope have been looking for evidence of suspected types of dark matter particles within faint dwarf galaxies near the Milky Way — relatively “boring” galaxies that have little activity but are known to contain large amounts of dark matter. The results?
These aren’t the particles we’re looking for.
80% of the material in the physical Universe is thought to be made of dark matter — matter that has mass and gravity but does not emit electromagnetic energy (and is thus invisible). Its gravitational effects can be seen, particularly in clouds surrounding galaxies where it is suspected to reside in large amounts. Dark matter can affect the motions of stars, galaxies and even entire clusters of galaxies… but when it all comes down to it, scientists still don’t really know exactly what dark matter is.
Possible candidates for dark matter are subatomic particles called WIMPs (Weakly Interacting Massive Particles). WIMPs don’t absorb or emit light and don’t interact with other particles, but whenever they interact with each other they annihilate and emit gamma rays.
If dark matter is composed of WIMPs, and the dwarf galaxies orbiting the Milky Way do contain large amounts of dark matter, then any gamma rays the WIMPs might emit could be detected by NASA’s Fermi Gamma-Ray Space Telescope.
After all, that’s what Fermi does.
Ten such galaxies — called dwarf spheroids — were observed by Fermi’s Large-Area Telescope (LAT) over a two-year period. The international team saw no gamma rays within the range expected from annihilating WIMPs were discovered, thus narrowing down the possibilities of what dark matter is.
“In effect, the Fermi LAT analysis compresses the theoretical box where these particles can hide,” said Jennifer Siegal-Gaskins, a physicist at the California Institute of Technology in Pasadena and a member of the Fermi LAT Collaboration.
[/caption]
So rather than a “failed experiment”, such non-detection means that for the first time researchers can be scientifically sure that WIMP candidates within a specific range of masses and interaction rates cannot be dark matter.
(Sometimes science is about knowing what not to look for.)
A paper detailing the team’s results appeared in the Dec. 9, 2011, issue of Physical Review Letters. Read more on the Fermi mission page here.