Could there be a mirror universe, where everything is backwards – and everybody has goatees? How badly do you need to bend the laws of physics to make this happen?
One of the great mysteries in cosmology is why the Universe is mostly matter and not antimatter. If you want to learn more about that specific subject, you can click here and watch an episode all about that.
During the Big Bang, nearly equal amounts of matter and antimatter were created, and subsequently annihilated. Nearly equal. And so we’re left with a Universe made of matter.
But could there be antimatter stars out there? With antimatter planets in orbit. Could there be a backwards Universe that operates just like our regular Universe, but everything’s made of antimatter? And if it’s out there, does it have to be evil? Do they only know how to conquer? Does everyone, even the antimatter babies and ladies, have handsome goatees? How about sashes? I hear they’re big on sashes. OOH and daggers. Gold daggers with little teensy antimatter emeralds and rubies.
Antimatter, without the goatee, was theorized in 1928 by Paul Dirac, who realized that one implication of quantum physics was that you could get electrons that had a positive charge instead of a negative charge. They were discovered by Carl D. Anderson just 4 years later, which he named “positron” for positive electron.
We believe he was clearly snubbing Dirac, by not naming them the “Diracitron”, alternately they were saving that name for a giant Japanese robot.
These antiparticles are created through high energy particle collisions happening naturally in the Universe, or unnaturally inside our “laugh in the face of God and nature” particle accelerators. We can even detect the annihilation out there in the Universe where matter and antimatter crash into each other.
Physicists have discovered a range of anti-particles. Anti-protons, anti-neutrons, anti-hydrogen, anti-helium. To date, there’s been no evidence of any goatees or sashes. Naturally, they wondered what might happen if the balance of the Universe was flipped. What if we had a Universe made out of mostly antimatter? Would it still… you know, work? Could you have antimatter stars, antimatter planets, and even those antimatter people we mentioned?
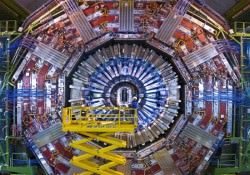
When physics swap out matter for anti-matter in their equations, they call it charge conjugation. It turns out, no. If you reversed the charge of all the particles in the Universe, it wouldn’t evolve in the same way as our “plain old non-sashed” Universe.
To fix this problem, physicists considered the implications if you had an actual mirror Universe, where all the particles behaved as if they were mirror images of themselves. This sounds a little more in line with our “Through a mirror, darkly” goatee and sash every day festival universe. This is all the bits backwards. Spin, charge, velocity, the works. They called this parity inversion. So, would this work?
Again, it turns out that the answer is no. It would almost work out, but there’s a tendency for the weak nuclear force, the one the governs nuclear decay to violate this idea of parity inversion. Even in a mirror Universe, the weak nuclear force is left-handed. Dammit, weak nuclear force, get your act together, if not just for the sake of the costumes and cooler bridge lighting.
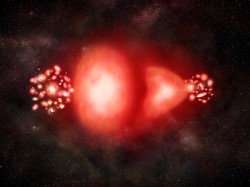
What if you reversed both the charge and the parity at the same time? What if you had antimatter in a mirror Universe? Physicists called this charge-parity symmetry, or CP symmetry.
In a dazzling experiment and absolute “what if” one-upmanship exercise by James Cronin and Val Fitch in 1964. They demonstrated that no, you can’t have a mirror-antimatter Universe evolve with our physical laws. This experiment won the Nobel Prize in 1980.
Physicists had one last trick up their sleeves. It turns out that if you reverse time itself as well as making everything out of antimatter and holding it up to a mirror, you get true symmetry. All the physical lays are preserved, and you’d get a Universe that would look exactly like our own.
It turns out we could live in a mirror Universe, as long as you were willing to reverse the charge of every particle and run time backwards. And if you did, it would be indistinguishable from the Universe we actually live in. Now, if you’ll excuse me, I think I need to call my tailor, I hear sashes are going to be huge this year.
So what do you think, do we live in the real Universe or the mirror Universe? Tell us in the comments below.