A Japanese telescope has produced our most detailed radio wave image yet of the Milky Way galaxy. Over a 3-year time period, the Nobeyama 45 meter telescope observed the Milky Way for 1100 hours to produce the map. The image is part of a project called FUGIN (FOREST Unbiased Galactic plane Imaging survey with the Nobeyama 45-m telescope.) The multi-institutional research group behind FUGIN explained the project in the Publications of the Astronomical Society of Japan and at arXiv.
The Nobeyama 45 meter telescope is located at the Nobeyama Radio Observatory, near Minamimaki, Japan. The telescope has been in operation there since 1982, and has made many contributions to millimeter-wave radio astronomy in its life. This map was made using the new FOREST receiver installed on the telescope.
When we look up at the Milky Way, an abundance of stars and gas and dust is visible. But there are also dark spots, which look like voids. But they’re not voids; they’re cold clouds of molecular gas that don’t emit visible light. To see what’s happening in these dark clouds requires radio telescopes like the Nobeyama.
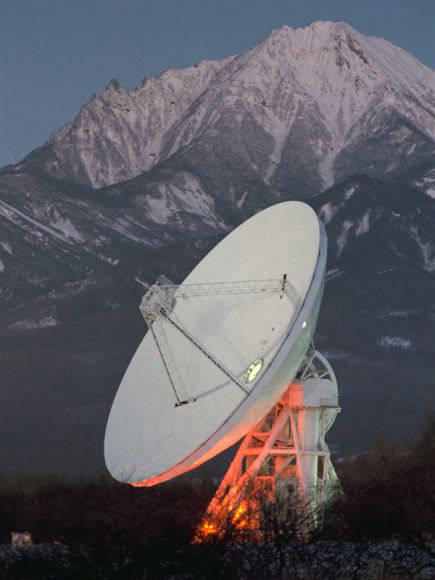
The Nobeyama was the largest millimeter-wave radio telescope in the world when it began operation, and it has always had great resolution. But the new FOREST receiver has improved the telescope’s spatial resolution ten-fold. The increased power of the new receiver allowed astronomers to create this new map.
The new map covers an area of the night sky as wide as 520 full Moons. The detail of this new map will allow astronomers to study both large-scale and small-scale structures in new detail. FUGIN will provide new data on large structures like the spiral arms—and even the entire Milky Way itself—down to smaller structures like individual molecular cloud cores.
FUGIN is one of the legacy projects for the Nobeyama. These projects are designed to collect fundamental data for next-generation studies. To collect this data, FUGIN observed an area covering 130 square degrees, which is over 80% of the area between galactic latitudes -1 and +1 degrees and galactic longitudes from 10 to 50 degrees and from 198 to 236 degrees. Basically, the map tried to cover the 1st and 3rd quadrants of the galaxy, to capture the spiral arms, bar structure, and the molecular gas ring.
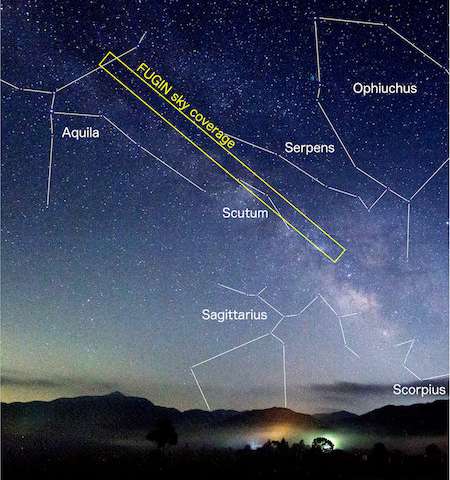
The aim of FUGIN is to investigate physical properties of diffuse and dense molecular gas in the galaxy. It does this by simultaneously gathering data on three carbon dioxide isotopes: 2CO, 13CO, and 18CO. Researchers were able to study the distribution and the motion of the gas, and also the physical characteristics like temperature and density. And the studying has already paid off.
FUGIN has already revealed things previously hidden. They include entangled filaments that weren’t obvious in previous surveys, as well as both wide-field and detailed structures of molecular clouds. Large scale kinematics of molecular gas such as spiral arms were also observed.
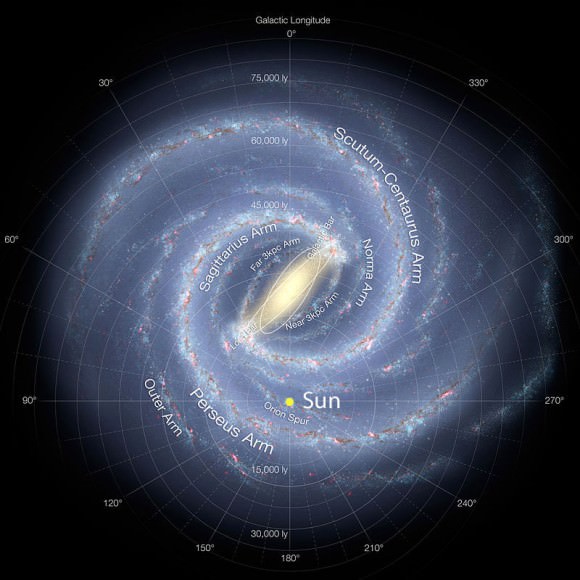
But the main purpose is to provide a rich data-set for future work by other telescopes. These include other radio telescopes like ALMA, but also telescopes operating in the infrared and other wavelengths. This will begin once the FUGIN data is released in June, 2018.
Millimeter wave radio astronomy is powerful because it can “see” things in space that other telescopes can’t. It’s especially useful for studying the large, cold gas clouds where stars form. These clouds are as cold as -262C (-440F.) At temperatures that low, optical scopes can’t see them, unless a bright star is shining behind them.
Even at these extremely low temperatures, there are chemical reactions occurring. This produces molecules like carbon monoxide, which was a focus of the FUGIN project, but also others like formaldehyde, ethyl alcohol, and methyl alcohol. These molecules emit radio waves in the millimeter range, which radio telescopes like the Nobeyama can detect.
The top-level purpose of the FUGIN project, according to the team behind the project, is to “provide crucial information about the transition from atomic gas to molecular gas, formation of molecular clouds and dense gas, interaction between star-forming regions and interstellar gas, and so on. We will also investigate the variation of physical properties and internal structures of molecular clouds in various environments, such as arm/interarm and bar, and evolutionary stage, for example, measured by star-forming activity.”
This new map from the Nobeyama holds a lot of promise. A rich data-set like this will be an important piece of the galactic puzzle for years to come. The details revealed in the map will help astronomers tease out more detail on the structures of gas clouds, how they interact with other structures, and how stars form from these clouds.