Since prehistoric times, human beings have looked up at at the night sky and pondered the mystery of the band of light that stretches across the heavens. And while theories have been advanced since the days of Ancient Greece as to what it could be, it was only with the birth of modern astronomy that scholars have come come to know precisely what it is – i.e. countless stars at considerable distances from Earth.
The term “Milky Way”, a term which emerged in Classical Antiquity to describe the band of light in the night sky, has since gone on to become the name for our galaxy. Like many others in the known Universe, the Milky Way is a barred, spiral galaxy that is part of the Local Group – a collection of 54 galaxies. Measuring 100,000 – 180,000 light-years in diameter, the Milky Way consists of between 100 and 400 billion stars.
Structure:
The Milky Way consists of a Galactic Center that is shaped like a bar and a Galactic Disk made up of spiral arms, all of which is surrounded by the Halo – which is made up of old stars and globular clusters. The Center, also known as “the bulge”, is a dense concentration of mostly old stars that measures about 10,000 light years in radius. This region is also the rotational center of the Milky Way.
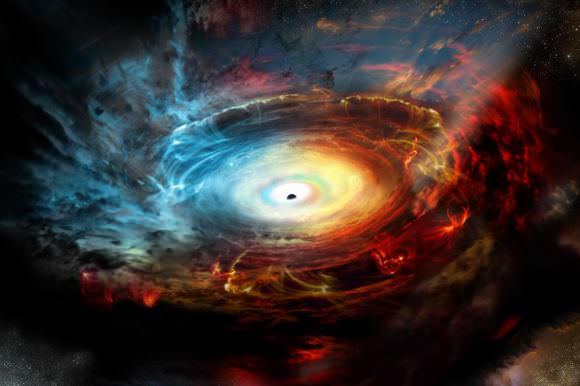
The Galactic Center is also home to an intense radio source named Sagittarius A*, which is believed to have a supermassive black hole (SMBH) at its center. The presence of this black hole has been discerned due to the apparent gravitational influence it has on surrounding stars. Astronomers estimate that it has a mass of between 4.1. and 4.5 million Solar masses.
Outside the barred bulge at the Galactic Center is the Galactic Disk of the Milky Way. This consists of stars, gas and dust which is organized into four spiral arms. These arms typically contain a higher density of interstellar gas and dust than the Galactic average, as well as a greater concentration of star formation. While there is no consensus on the exact structure or extent of these spiral arms, they are commonly grouped into two or four different arms.
In the case of four arms, this is based on the traced paths of gas and younger stars in our galaxy, which corresponds to the Perseus Arm, the Norma and Outer Arm, the Scutum-Centaurum Arm, and the Carina-Sagittarius Arm. There are also at least two smaller arms, which include the Cygnus Arm and the Orion Arm. Meanwhile, surveys based on the presence of older stars show only two major spirals arms – the Perseus arm and the Scutum–Centaurus arm.
Beyond the Galactic Disk is the Halo, which is made up of old stars and globular clusters – 90% of which lie within 100,000 light-years (30,000 parsecs) from the Galactic Center. Recent evidence provided by X-ray observatories indicates that in addition to this stellar halo, the Milky way also has a halo of hot gas that extends for hundreds of thousands of light years.
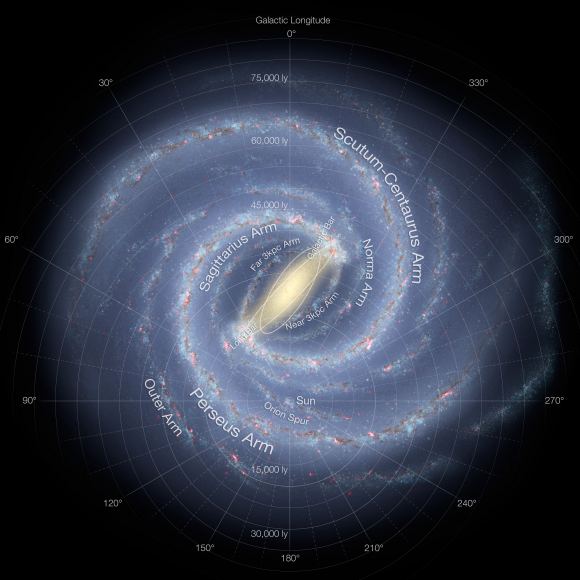
Size and Mass:
The Galactic Disk of the Milky Way Galaxy is approximately 100,000 light years in diameter and about 1,000 light years thick. It is estimated to contain between 100 and 400 billion stars, though the exact figure depends on the number of very low-mass M-type (aka. red dwarf) stars. This is difficult to determine because these stars also have low-luminosity compared to other class.
The distance from the Sun to the Galactic Center is estimated to be between 25,000 to 28,000 light years (7,600 to 8,700 parsecs). The Galactic Center’s bar (aka. its “bulge”) is thought to be about 27,000 light-years in length and is composed primarily of red stars, all of which are thought to be ancient. The bar is surrounded by the ‘5-kpc ring’, a region that contains much of the galaxy’s molecular hydrogen and where star-formation is most intense.
The Galactic Disk has a diameter of between 70,000 and 100,000 light-years. It does not have a sharp edge, a radius beyond which there are no stars. However, the number of stars drops slowly with distance from the center. Beyond a radius of roughly 40,000 light years, the number of stars drops much faster the farther you get from the center.
Location of the Solar System:
The Solar System is located near the inner rim of the Orion Arm, a minor spiral arm located between the Carina–Sagittarius Arm and the Perseus Arm. This arm measures some 3,500 light-years (1,100 parsecs) across, approximately 10,000 light-years (3,100 parsecs) in length, and is at a distance of about 25,400 to 27,400 light years (7.78 to 8.4 thousand parsecs) from the Galactic Center.
History of Observation:
Our galaxy was named because of the way the haze it casts in the night sky resembled spilled milk. This name is also quite ancient. It is translation from the Latin “Via Lactea“, which in turn was translated from the Greek for Galaxias, referring to the pale band of light formed by stars in the galactic plane as seen from Earth.
Persian astronomer Nasir al-Din al-Tusi (1201–1274) even spelled it out in his book Tadhkira: “The Milky Way, i.e. the Galaxy, is made up of a very large number of small, tightly clustered stars, which, on account of their concentration and smallness, seem to be cloudy patches. Because of this, it was likened to milk in color.”
Astronomers had long suspected the Milky Way was made up of stars, but it wasn’t proven until 1610, when Galileo Galilei turned his rudimentary telescope towards the heavens and resolved individual stars in the band across the sky. With the help of telescopes, astronomers realized that there were many, many more stars in the sky, and that all of the ones that we can see are a part of the Milky Way.
In 1755, Immanuel Kant proposed that the Milky Way was a large collection of stars held together by mutual gravity. Just like the Solar System, this collection would be rotating and flattened out as a disk, with the Solar System embedded within it. Astronomer William Herschel (discoverer of Uranus) tried to map its shape in 1785, but he didn’t realize that large portions of the galaxy are obscured by gas and dust, which hide its true shape.
It wasn’t until the 1920s, when Edwin Hubble provided conclusive evidence that the spiral nebulae in the sky were actually whole other galaxies, that the true shape of our galaxy was known. Thenceforth, astronomers came to understand that the Milky Way is a barred, spiral galaxy, and also came to appreciate how big the Universe truly is.
The Milky Way is appropriately named, being the vast and cloudy mass of stars, dust and gas it is. Like all galaxies, ours is believed to have formed from many smaller galaxies colliding and combining in the past. And in 3 to 4 billion years, it will collide with the Andromeda Galaxy to form an even larger mass of stars, gas and dust. Assuming humanity still exists by then (and survives the process) it should make for some interesting viewing!
We have written many interesting articles about the Milky Way here at Universe Today. Here’s 10 Interesting Facts About the Milky Way, How Big is the Milky Way?, Why is our Galaxy Called the Milky Way?, What is the Closest Galaxy to the Milky Way?, Where is the Earth in the Milky Way?, The Milky Way has Only Two Spiral Arms, and It’s Inevitable: Milky Way, Andromeda Galaxy Heading for Collision.
If you’d like more info on galaxies, check out Hubblesite’s News Releases on Galaxies, and here’s NASA’s Science Page on Galaxies.
We’ve also recorded an episode of Astronomy Cast about the Milky Way. Listen here, Episode 99: The Milky Way.
Sources: