Is our 13.8 billion year old universe actually in its death throes?
Poor Universe, its demise announced right in it’s prime. At only 13.8 billion years old, when you peer across the multiverse it’s barely middle age. And yet, it sadly dwindles here in hospice.
Is it a Galactus infestation? The Unicronabetes? Time to let go, move on and find a new Universe, because this one is all but dead and gone and but a shell of its former self.
The news of imminent demise was recently broadcast in mid 2015. Based on research looking at the light coming from over 200,000 galaxies, they found that the galaxies are putting out half as much light as they were 2 billion years ago. So if our math is right, less light equals more death.
So tell it to me straight, Doctor Spaceman(SPAH-CHEM-AN), how long have we got? Astronomers have known for a long time that the Universe was much more active in the distant past, when everything was closer and denser, and better. Back then, more of it was the primordial hydrogen left over from the Big Bang, supplying galaxies for star formation. Currently, there are only 1 to 3 new stars formed in the Milky Way every year. Which is pretty slow by Milky Way standards.
Not even at the busiest time of star formation, our Sun formed 5 billion years ago. 5 billion years before that, just a short 4 billion after the Big Bang, star formation peaked out. There were 30 times more stars forming then, than we see today.
When stars were formed actually makes a difference. For example, the fact that it took so long for our Sun to form is a good thing. The heavier elements in the Solar System, really anything higher up the periodic table from hydrogen and helium, had to be formed inside other stars. Main sequence stars like our own Sun spew out heavier elements from their solar winds, while supernovae created the heaviest elements in a moment of catastrophic collapse. Astronomers are pretty sure we needed a few generations of stars to build up enough of the heavier elements that life depends on, and probably wouldn’t be here without it.
Even if life did form here on Earth billions of years ago, when the Universe was really cranking, it would wish it was never born. With 30 times as much star formation going on, there would be intense radiation blasting away from all these newly forming stars and their subsequent supernovae detonations. So be glad life formed when it did. Sometimes a little quiet is better.
So, how long has the Universe got? It appears that it’s not going to crash together in the future, it’s just going to keep on expanding, and expanding, forever and ever.
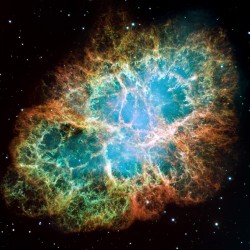
In a few billion years, star formation will be a fraction of what it is today. In a few trillion, only the longest lived, lowest mass red dwarfs will still be pushing out their feeble light. Then, one by one, galaxies will see their last star flicker and fade away into the darkness. Then there’ll only be dead stars and dead planets, cooling down to the background temperature of the Universe as their galaxies accelerate from one another into the expanding void.
Eventually everything will be black holes, or milling about waiting to be trapped in black holes. And these black holes themselves will take an incomprehensible mighty pile of years to evaporate away to nothing.
So yes, our Universe is dying. Just like in a cheery Sartre play, it started dying the moment it began its existence. According to astronomers, the Universe will never truly die. It’ll just reach a distant future when there’s so little usable energy, it’ll be mostly dead. Dead enough? Dead inside.
As Miracle Max knows, mostly dead is still slightly alive. Who knows what future civilizations will figure out in the googol years between then and now.
Too sad? Let’s wildly speculate on futuristic technologies advanced civilizations will use to outlast the heat death of the Universe or flat out cheat death and re-spark it into a whole new cycle of Universal renewal.