Most of the exoplanets we’ve discovered orbit red dwarf stars. This isn’t because red dwarfs are somehow special, simply that they are common. About 75% of the stars in the Milky Way are red dwarfs, so you would expect red dwarf planets to be the most abundant. This also means that most habitable worlds are going to orbit these small, cool stars, and that has some significant consequences for our search for life.
Continue reading “Exoplanets Could be Hiding Their Atmospheres”TRAPPIST-1 Outer Planets Likely Have Water

The TRAPPIST-1 solar system generated a swell of interest when it was observed several years ago. In 2016, astronomers using the Transiting Planets and Planetesimals Small Telescope (TRAPPIST) at La Silla Observatory in Chile detected two rocky planets orbiting the red dwarf star, which took the name TRAPPIST-1. Then, in 2017, a deeper analysis found another five rocky planets.
It was a remarkable discovery, especially because up to four of them could be the right distance from the star to have liquid water.
Continue reading “TRAPPIST-1 Outer Planets Likely Have Water”Is the JWST Now an Interplanetary Meteorologist?
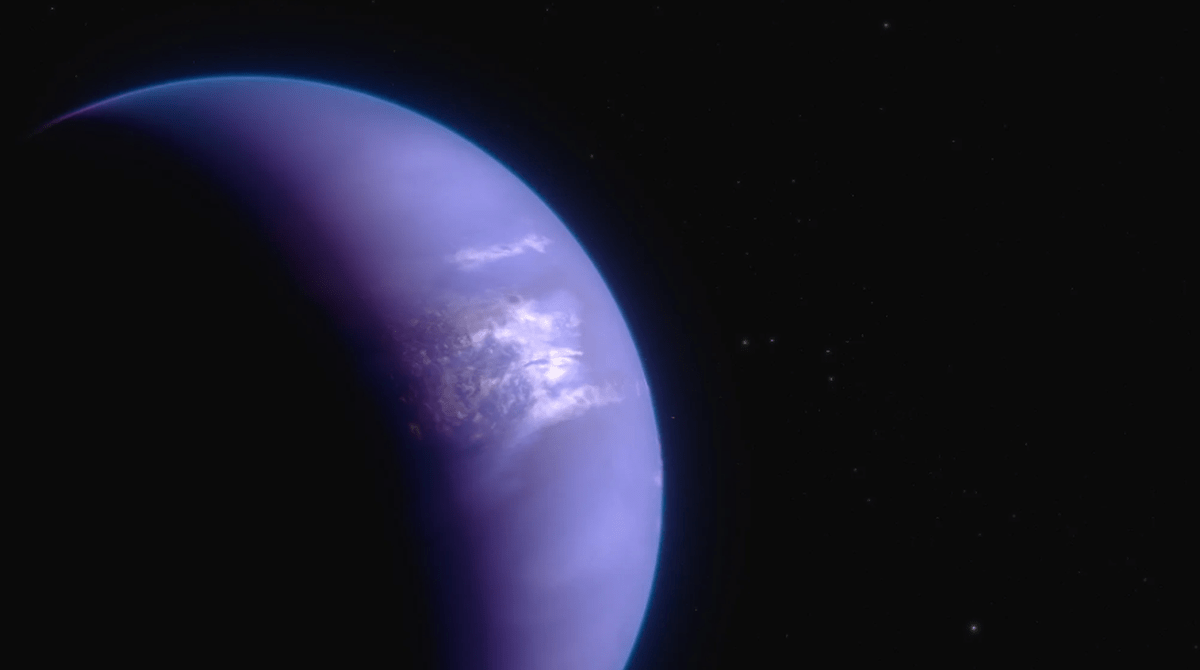
The JWST keeps one-upping itself. In the telescope’s latest act of outdoing itself, it examined a distant exoplanet to map its weather. The forecast?
An unending, blistering inferno driven by ceaseless supersonic winds.
Continue reading “Is the JWST Now an Interplanetary Meteorologist?”Venus' Atmosphere Stops it From Locking to the Sun
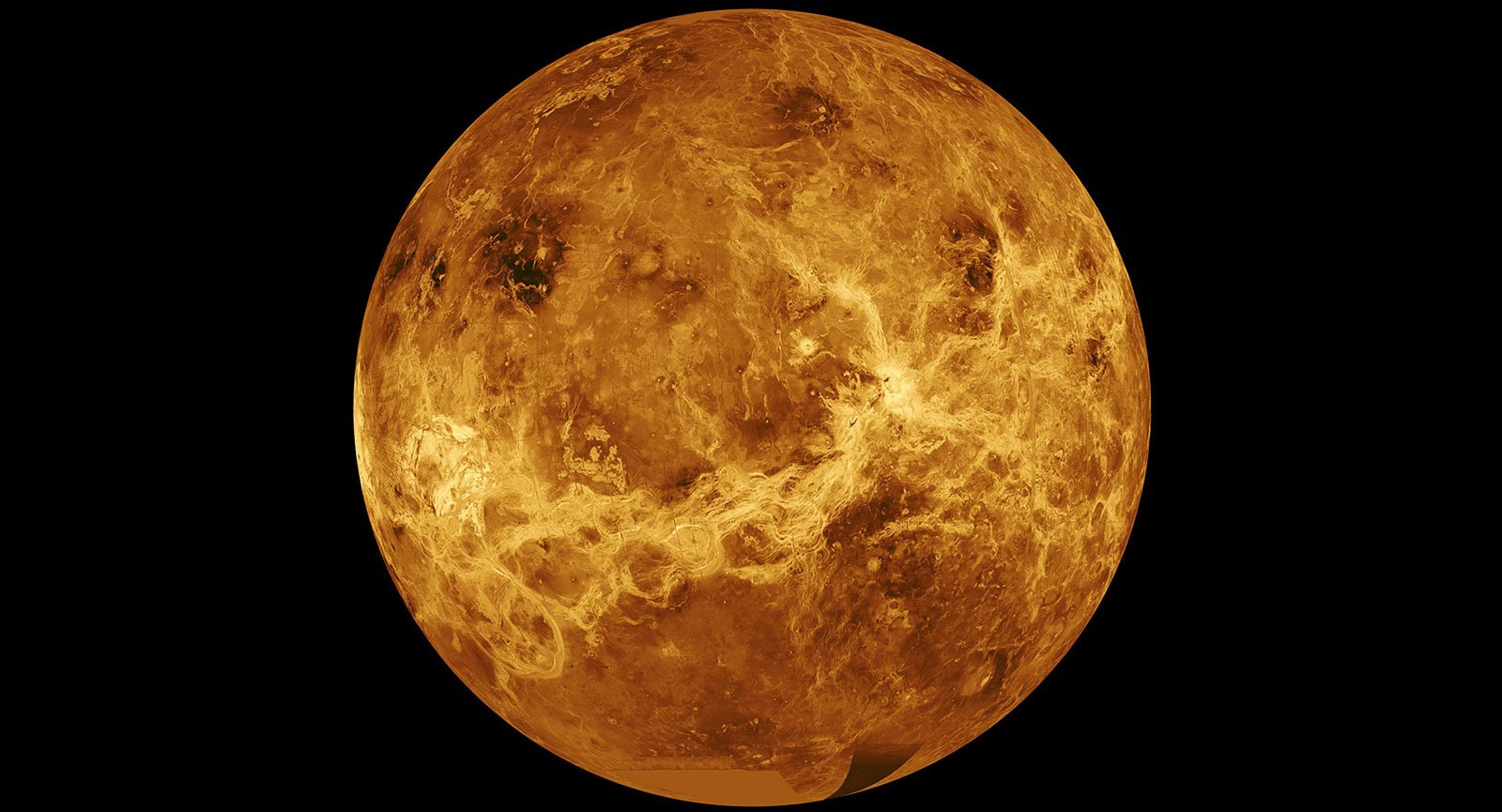
Of the thousands of exoplanets we’ve discovered, most of them closely orbit red dwarf stars. Part of this is because planets with short orbital periods are easier to find, but part of this is that red dwarf stars make up about 75% of the stars in our galaxy. This propensity of close orbiting planets has some pretty big implications for “potentially habitable” worlds, not the least of which is that most of these planets are likely tidally locked to their star. Or so we’ve thought.
Continue reading “Venus' Atmosphere Stops it From Locking to the Sun”Your Umbrella is Insufficient on a Planet Where it Rains Iron
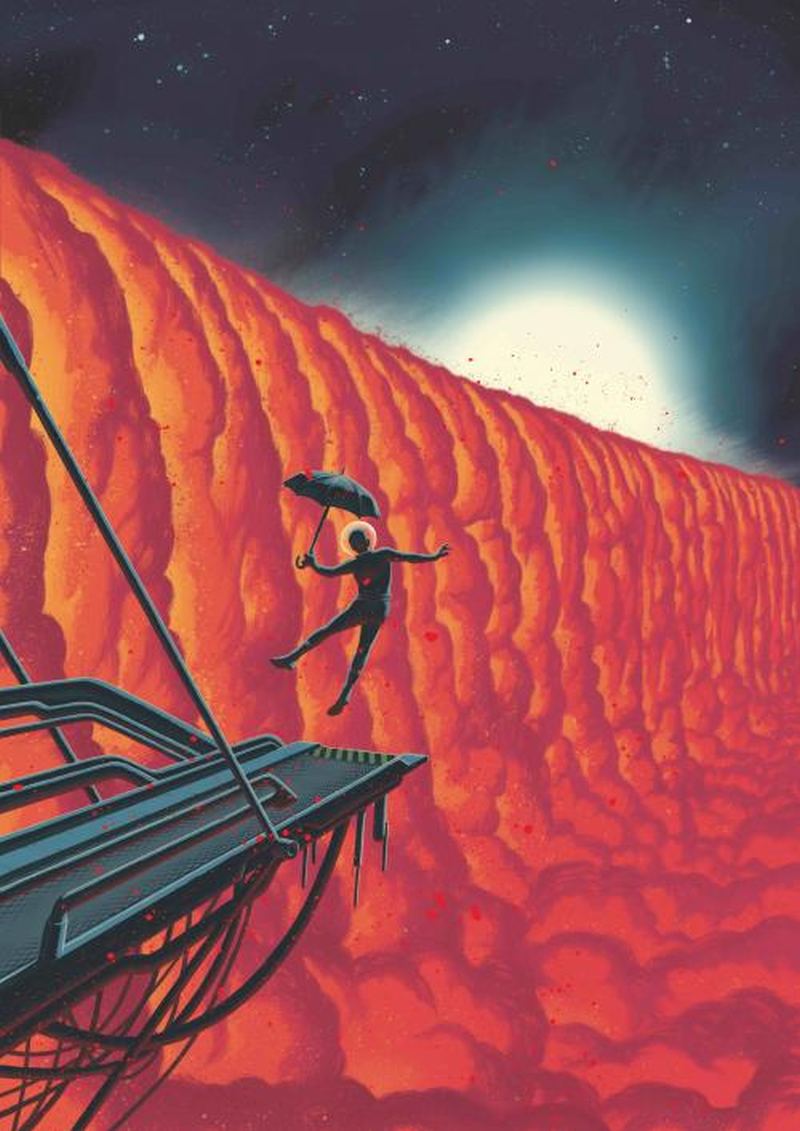
Imagine a planet where it rained iron. Sounds impossible. But on one distant exoplanet, which is tidally locked to its star, the nightside has to contend with a ferrous downpour.
Continue reading “Your Umbrella is Insufficient on a Planet Where it Rains Iron”When Will Earth Lock to the Moon?
We always see the same side of the Moon. It’s always up there, staring down at us with its terrifying visage. Or maybe it’s a creepy rabbit? Anyway, it’s always showing us the same face, and never any other part.
This is because the Moon is tidally locked to the Earth; the same fate that affects every single large moon orbiting a planet. The Moon is locked to the Earth, the Jovian moons are locked to Jupiter, Titan is locked to Saturn, etc.
As the Moon orbits the Earth, it slowly rotates to keep the same hemisphere facing us. Its day is as long as its year. And standing on the surface of the Moon, you’d see the Earth in roughly the same spot in the sky. Forever and ever.
We see this all across the Solar System.
But there’s one place where this tidal locking goes to the next level: the dwarf planet Pluto and its large moon Charon are tidally locked to each other. In other words, the same hemisphere of Pluto always faces Charon and vice versa.
It take Pluto about 6 and a half days for the Sun to return to the same point in the sky, which is the same time it takes Charon to complete an orbit, which is the same time it takes the Sun to pass through the sky on Charon.
Since Pluto eventually locked to its moon, can the same thing happen here on Earth. Will we eventually lock with the Moon?
Before we answer this question, let’s explain what’s going on here. Although the Earth and the Moon are spheres, they actually have a little variation. The gravity pulling on each world creates love handle tidal bulges on each world.
And these bulges act like a brake, slowing down the rotation of the world. Because the Earth has 81 times the mass of the Moon, it was the dominant force in this interaction.
In the early Solar System, both the Earth and the Moon rotated independently. But the Earth’s gravity grabbed onto those love handles and slowed down the rotation of the Moon. To compensate for the loss of momentum in the system, the Moon drifted away from the Earth to its current position, about 370,000 kilometers away.
But Moon has the same impact on the Earth. The same tidal forces that cause the tides on Earth are slowing down the Earth’s rotation bit by bit. And the Moon is continuing to drift away a few centimeters a year to compensate.
It’s hard to estimate exactly when, but over the course of tens of billions of years, the Earth will become locked to the Moon, just like Pluto and Charon.
Of course, this will be long after the Sun has died as a red giant. And there’s no way to know what kind of mayhem that’ll cause to the Earth-Moon system. Other planets in the Solar System may shift around, and maybe even eject the Earth into space, taking the Moon with it.
What about the Sun? Is it possible for the Earth to eventually lock gravitationally to the Sun?
Astronomers have found extrasolar planets orbiting other stars which are tidally locked. But they’re extremely close, well within the orbit of Mercury.
Here in our Solar System, we’re just too far away from the Sun for the Earth to lock to it. The gravitational influence of the other planets like Venus, Mars and Jupiter perturb our orbit and keep us from ever locking. Without any other planets in the Solar System, though, and with a Sun that would last forever, it would be an inevitability.
It is theoretically possible that the Earth will tidally lock to the Moon in about 50 billion years or so. Assuming the Earth and Moon weren’t consumed during the Sun’s red giant phase. I guess we’ll have to wait and see.
What is Tidal Locking?
The Moon is tidally locked to the Earth, which means that it always shows one face to our planet. In fact, this is the case for most the large moons in the Solar System. What’s the process going on to make this happen?
Just look at the Moon, isn’t it beautiful? Take out a nice pair of binoculars, or a small telescope tonight and you’ll be able to see huge craters and ancient lava plains. Look again tomorrow, and you’ll be able to see… the exact same things. As you know, our modest Moon only shows us one face. Ever.
If you could look at the Moon orbiting the Earth from above, you’d see that it orbits once on its axis exactly as long as it takes to orbit once around our planet. It’s always turning, showing us exactly the same face. What’s it hiding?
The Moon isn’t the only place in the Solar System where this happens. All major moons of Jupiter and Saturn show the same face to their parent. Pluto and Charon are even stranger, the two worlds are locked, facing one another for all eternity. Astronomers call this tidal locking, and happens because of the gravitational interaction between worlds.
As you’re aware, the Moon is pulling at the Earth, causing the tides. In fact, the pull of the Moon is so strong that the ground itself rises up 30 cm, about a foot, as it passes by.
It’s even more powerful on the Moon. The gravity from the Earth distorts the Moon into an oblong shape. The sides pointed towards and away from the Earth bulge outward, while the others are pulled inward to compensate. It makes the Moon football shaped.
It’s no big deal now, but in the ancient past, shortly after its formation, the Moon was spinning rapidly. This meant that the part of the Moon bulged towards us was changing constantly, like water tides on Earth.
Vast amounts of rock need to shift and change shape to bulge towards the Earth and then settle down again, and this takes time. The position of the bulges on the Moon were always a little out of alignment with the pull of gravity of the Earth.
These bulges acted like handles that the Earth’s gravity could grab onto, and torque it back into place. Over time, the Earth’s gravity slowed down the rotation speed of the Moon until it stopped, forever.
This same process happened on all the large moons in the Solar System.
Because of its smaller mass, our Moon became tidally locked to the Earth billions of years ago. Now the process is continuing to make the Earth tidally locked to the Moon as well.
In the distant distant future, the Moon will stop moving in the sky, and hang motionless, visible from only half the Earth.
How distant? In about 50 billion years, long after the Sun has died, the Earth and the Moon will finally be tidally locked to each other, just like Romeo and Juliet, Fry and Leela, Pluto and Charon. The force of gravity is a powerful thing. Powerful enough to stop a moon in its tracks.
Did you have any other questions about the Moon? Post your suggestions in the comments and we’d be glad to make more videos and dig deeper!
How Long is a Day on Earth?
I’m going to ask you how long a day is on Earth, and you’re going to get the haunting suspicion that this is a trap. Your instincts are right, it’s a trap! The answer may surprise you.
How long is a day on Earth? Or more specifically, how long does it take for the Earth to turn once on its axis? For all the stars to move through the sky and return to their original position? Go ahead, and yell your answer answer at the screen… 24 hours?
Wrong! It only takes 23 hours, 56 minutes and 4.0916 seconds for the Earth to turn once its axis. Unless that’s what you said. In which case, congratulations!
I’m sure you’re now stumbling around in an incoherent state, trying to understand how you could have possibly messed this up. Were you reprogrammed by the hidden chronology conspiracy? Have time travellers been setting back all your clocks every day by 4 minutes? How was your whole life a lie?
Here’s the deal. When you consider a day, you’re probably thinking of your trusty clock, or maybe that smartphone lock screen that clearly measures 24 hours.
What you have come to understand as a “day” is classified by astronomers as a solar day. It’s the amount of time it takes for the Sun to move through the sky and return to roughly the same spot.
This is different from the amount of time it takes for the Earth to turn once on its axis – the 23 hours, 56 minutes. Also known as a sidereal day.
Why are these two numbers different? Imagine the Earth orbiting the Sun, taking a full 365 days, 5 hours, 48 minutes and 46 seconds to complete the entire journey. At the same time, the Earth is spinning on its axis.
Each day that goes by, the Earth needs to turn a little further for the Sun to return to the same place in the sky.… And that extra time is about 4 minutes.
If we only measured sidereal days, the position of the Sun would slip back, day after day. For half of the year, the Sun would be up between 12am and 12pm, and for the other half, it would be between 12pm and 12am. There would be no connection between what time it is, and whether or not the Sun is in the sky.
Can you imagine teaching your children how to read a clock, and then getting them to multiply that by the calendar to figure out when My Little Pony: Friendship is Magic starts? Madness.
Better to keep them in the dark, teach them that a day is 24 hours, and deny all knowledge when they get a little older, and start to ask you challenging questions. But pedants among you already knew that, didn’t you?
You already knew that a sidereal day is a little shorter than a solar day, and that everyone else has been living a lie. You’re the only one who can read the signs and know the terrifying truth. Aren’t you? Well, I’m here to tell you that you’re wrong too. There’s a deeper conspiracy that you’re not a part of. Dear Pedant, your life is also a lie.
The axis of the Earth’s pole, the imaginary line that you could draw between the south pole and the north pole is currently pointed roughly at Polaris, aka The North Star. But we’re wobbling like a top, and where the axis is pointing is slowly precessing westward over the course of 26,000 years. This means that a sidereal day is actually 0.0084 seconds shorter when you account for this extra movement of the Earth’s axis.
There are other events that can increase or decrease the length of an Earth day. Because of our tidal interactions with the Moon, the length of a day on Earth has increased by about 1.7 milliseconds over the last 100 years. Powerful earthquakes can change the Earth’s rotation time by a few microseconds depending on how the tectonic plates shove around. Even as the glaciers melt, the rotation speed slows down a little more.
So, if someone asks you how long a day is, make sure they clarify whether it’s a solar day or a sidereal day. And then ask if they’d like you to incorporate the Earth’s precession, tidal locking and recent earthquakes into the calculation.
If they give you a knowing nod, congratulations, you’re talking to another member of the vast chronology conspiracy.
When did you discover your whole life was a lie? Tell us in the comments below.
Oct. 7, 1959 – Our First Look at the Far Side of the Moon
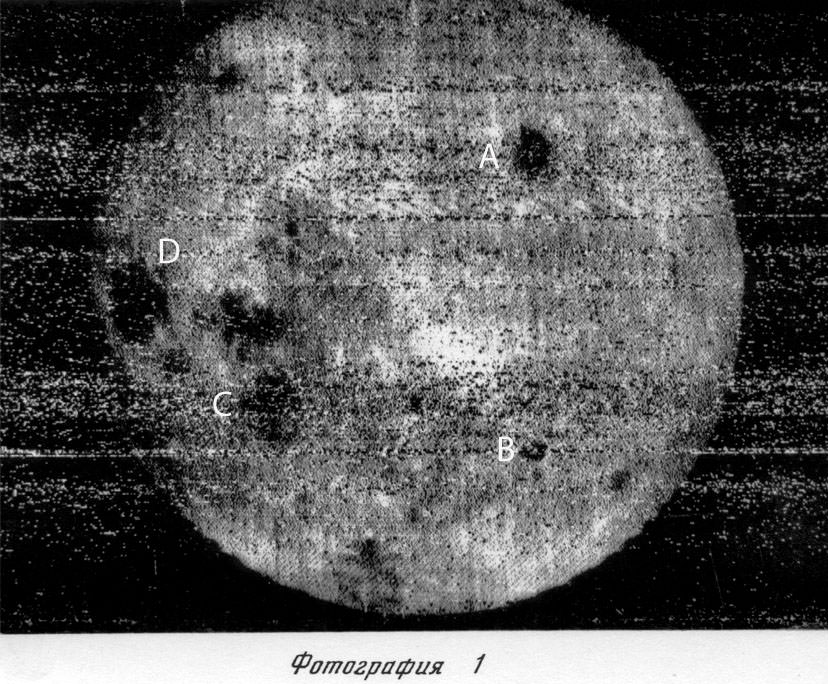
For millennia, human eyes have seen only one face of the moon. Put a dude from the Iron Age in a time machine and whisk him to 2013 and he’d see the same pattern of light lunar highlands punctuated by dark grey spots you see. Night after night after night.
That all changed 54 years ago today when the Soviet Union’s Luna 3 probe opened its camera shutter and snapped the first pictures of the lunar far side. Though blurry and banded with electronic noise, everyone who saw them sat up in surprise. The backside barely resembled the front. It lacked in the familiar lunar maria, the dark spots that we instinctively patch together to form the face of the “man in the moon”.
Only two dark ovals were seen, Mare Moscoviense (Sea of Moscow) and the lava-filled floor of the crater Tsiolkovsky, named for Konstantin Tsiolkovksy, the Russian rocket pioneer. The rest, which looks like dried paste, is jammed with craters and related the near side’s light-toned, cratered highlands. Both are remnants of the original lunar crust that solidified as the moon cooled after formation.
Darker areas or “seas” are more recent basaltic lavas that welled up to fill huge impact scars left by colliding asteroids. They contain iron-rich minerals from deep beneath the crust which make them less reflective, hence darker in comparison to the highlands.
The moon hides its back or far side through a neat trick – it rotates at the same rate as it revolves around the Earth. Normally, rotation would bring new features into view, but every little bit it turns, it moves an equal amount along its orbit, hiding what would otherwise be exposed. It’s called synchronous rotation or tidal locking. Most of the larger moons in the solar system are tidally locked to their planets. Jupiter’s four biggest and brightest moons are a great example.
Equipped with both wide angle (200 mm) and telephoto (500 mm) lenses, Luna 3 took 29 pictures covering about 70 percent of the far side during its loop around the moon. The first picture was shot from 39,500 miles away (63,500 km), the last taken 40 minutes later from 41,445 miles (66,700 km) distant. After the photo session was done, the probe passed over the moon’s north pole and headed back toward Earth.
Temperature and radiation-resistant film used for the photos was automatically moved to an onboard processor where it was developed, fixed and dried. A cathode ray tube then shot a beam of light through the film and onto a photoelectric multiplier, a light-sensitive device that converted the different gradations of tone into electric signals which were then transmitted to Earth. Almost sounds like a fire brigade, but hey it worked!
So what’s the reason for the moon’s split personality? We know the far side crust is 50 miles (80 km) thick versus 37 miles (60 km) for the near side. A thicker far side crust may have prevented magma from reaching and flooding the surface as they did on the near side. Heat released by the decay of radioactive elements also may play a role. NASA’s Lunar Prospector probe found more on the near side, where they may have encouraged the formation of hot magmas that eventually found their way to the surface.
What caused the fascinating asymmetry is unknown, but it may have to do with the slowing of the moon’s rotation into its present tidally-locked state under the heavy hand of Earth’s dominating gravitational influence.