An undersea volcano erupted near the Pacific island of Tonga, and several satellites caught the incredible explosion in action. The blast of the Hunga Tonga Hunga Ha’apai volcano created a plume of ash, steam and gas mushrooming above the Pacific Ocean, with a quickly expanding shockwave visible from orbit. Japan’s Himawari-8 weather satellite recorded this dramatic video:
Continue reading “Tonga’s Incredible Underwater Volcano Eruption Seen From Space”40 Years Ago, Mount St. Helens Blew its Top Off
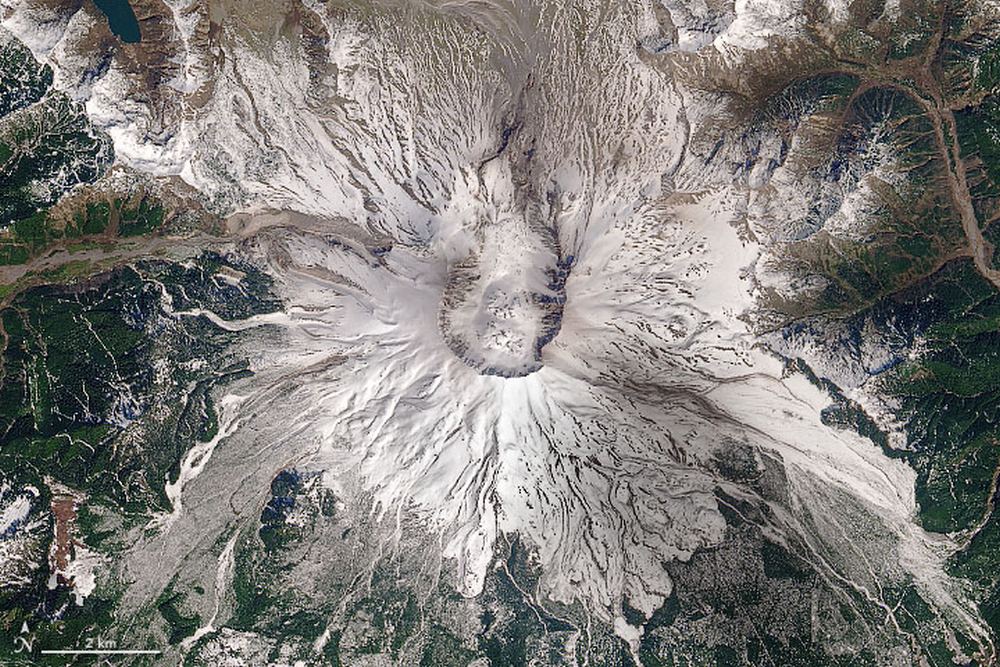
One day, my Grade Nine science class got way more interesting.
Suddenly, volcanoes weren’t just something in textbooks. Though I was in neighbouring British Columbia when Mt. St. Helens erupted, there was still a layer of ash on our cars and everything else. For a teenager with a burgeoning interest in science, it was awesome.
Continue reading “40 Years Ago, Mount St. Helens Blew its Top Off”Anak Krakatau Erupted a Few Days Ago. Here’s What it Looked Like From Space
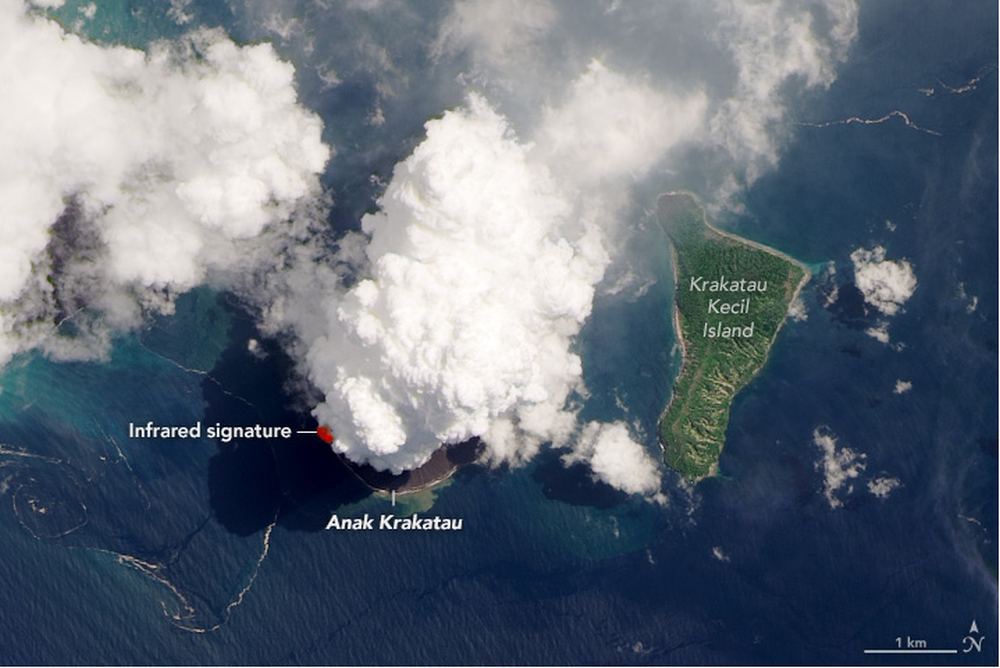
In between the Indonesian islands of Java and Sumatra lies the Sunda Strait. And in the Sunda Strait lies the much smaller island of Anak Krakatau, one of Earth’s active volcanoes. It’s erupted more than 50 times in the past 2,000 years, and now it’s doing it again.
Continue reading “Anak Krakatau Erupted a Few Days Ago. Here’s What it Looked Like From Space”Eruption of the Raikoke Volcano, Seen From Space
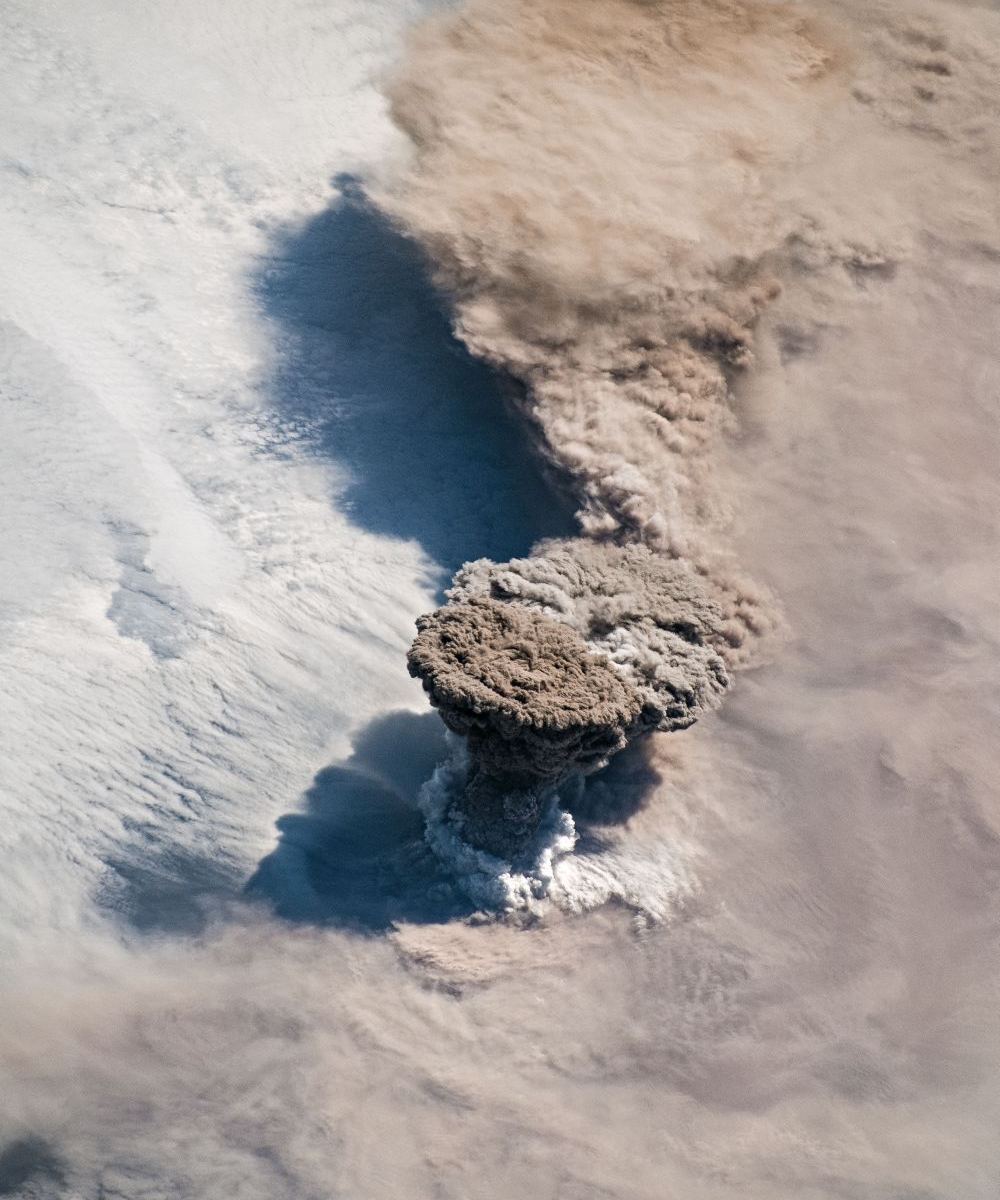
The Raikoke Volcano, dormant for a very long time, has awoken from its slumber. The volcanic island is in the Kuril Island chain, near the Kamchatka Peninsula in Russia. Unlike its more volcanically active neighbours, Raikoke has been dormant since 1924.
Thanks to astronauts on the International Space Station, we have gorgeous photos of the eruption.
Continue reading “Eruption of the Raikoke Volcano, Seen From Space”What About a Mission to Titan?
As you probably know, NASA recently announced plans to send a mission to Jupiter’s moon Europa. If all goes well, the Europa Clipper will blast off for the world in the 2020s, and orbit the icy moon to discover all its secrets.
And that’s great and all, I like Europa just fine. But you know where I’d really like us to go next? Titan.
Titan, as you probably know, is the largest moon orbiting Saturn. In fact, it’s the second largest moon in the Solar System after Jupiter’s Ganymede. It measures 5,190 kilometers across, almost half the diameter of the Earth. This place is big.
It orbits Saturn every 15 hours and 22 days, and like many large moons in the Solar System, it’s tidally locked to its planet, always showing Saturn one side.
Before NASA’s Voyager spacecraft arrived in 1980, astronomers actually thought that Titan was the biggest moon in the Solar System. But Voyager showed that it actually has a thick atmosphere, that extends well into space, making the true size of the moon hard to judge.
This atmosphere is one of the most interesting features of Titan. In fact, it’s the only moon in the entire Solar System with a significant atmosphere. If you could stand on the surface, you would experience about 1.45 times the atmospheric pressure on Earth. In other words, you wouldn’t need a pressure suit to wander around the surface of Titan.
You would, however, need a coat. Titan is incredibly cold, with an average temperature of almost -180 Celsius. For you Fahrenheit people that’s -292 F. The coldest ground temperature ever measured on Earth is almost -90 C, so way way colder.
You would also need some way to breathe, since Titan’s atmosphere is almost entirely nitrogen, with trace amounts of methane and hydrogen. It’s thick and poisonous, but not murderous, like Venus.
Titan has only been explored a couple of times, and we’ve actually only landed on it once.
The first spacecraft to visit Titan was NASA’s Pioneer 11, which flew past Saturn and its moons in 1979. This flyby was followed by NASA’s Voyager 1 in 1980 and then Voyager 2 in 1981. Voyager 1 was given a special trajectory that would take it as close as possible to Titan to give us a close up view of the world.
Voyager was able to measure its atmosphere, and helped scientists calculate Titan’s size and mass. It also got a hint of darker regions which would later turn out to be oceans of liquid hydrocarbons.
The true age of Titan exploration began with NASA’s Cassini spacecraft, which arrived at Saturn on July 4, 2004. Cassini made its first flyby of Titan on October 26, 2004, getting to within 1,200 kilometers or 750 miles of the planet. But this was just the beginning. By the end of its mission later this year, Cassini will have made 125 flybys of Titan, mapping the world in incredible detail.
Cassini saw that Titan actually has a very complicated hydrological system, but instead of liquid water, it has weather of hydrocarbons. The skies are dotted with methane clouds, which can rain and fill oceans of nearly pure methane.
And we know all about this because of Cassini’s Huygen’s lander, which detached from the spacecraft and landed on the surface of Titan on January 14, 2005. Here’s an amazing timelapse that shows the view from Huygens as it passed down through the atmosphere of Titan, and landed on its surface.
Huygens landed on a flat plain, surrounded by “rocks”, frozen globules of water ice. This was lucky, but the probe was also built to float if it happened to land on liquid instead.
It lasted for about 90 minutes on the surface of Titan, sending data back to Earth before it went dark, wrapping up the most distant landing humanity has ever accomplished in the Solar System.
Although we know quite a bit about Titan, there are still so many mysteries. The first big one is the cycle of liquid. Across Titan there are these vast oceans of liquid methane, which evaporate to create methane clouds. These rain, creating mists and even rivers.
Is it volcanic? There are regions of Titan that definitely look like there have been volcanoes recently. Maybe they’re cryovolcanoes, where the tidal interactions with Saturn cause water to well up from beneath crust and erupt onto the surface.
Is there life there? This is perhaps the most intriguing possibility of all. The methane rich system has the precursor chemicals that life on Earth probably used to get started billions of years ago. There’s probably heated regions beneath the surface and liquid water which could sustain life. But there could also be life as we don’t understand it, using methane and ammonia as a solvent instead of water.
To get a better answer to these questions, we’ve got to return to Titan. We’ve got to land, rove around, sail the oceans and swim beneath their waves.
Now you know all about this history of the exploration of Titan. It’s time to look at serious ideas for returning to Titan and exploring it again, especially its oceans.
Planetary scientists have been excited about the exploration of Titan for a while now, and a few preliminary proposals have been suggested, to study the moon from the air, the land, and the seas.
First up, there’s the Titan Saturn System Mission, a mission proposed in 2009, for a late 2020s arrival at Titan. This spacecraft would consist of a lander and a balloon that would float about in the atmosphere, and study the world from above. Over the course of its mission, the balloon would circumnavigate Titan once from an altitude of 10km, taking incredibly high resolution images. The lander would touch down in one of Titan’s oceans and float about on top of the liquid methane, sampling its chemicals.
As we stand right now, this mission is in the preliminary stages, and may never launch.
In 2012, Dr. Jason Barnes and his team from the University of Idaho proposed sending a robotic aircraft to Titan, which would fly around in the atmosphere photographing its surface. Titan is actually one of the best places in the entire Solar System to fly an airplane. It has a thicker atmosphere and lower gravity, and unlike the balloon concept, an airplane is free to go wherever it needs powered by a radioactive thermal generator.
Although the mission would only cost about $750 million or so, NASA hasn’t pushed it beyond the conceptual stage yet.
An even cooler plan would put a boat down in one of Titan’s oceans. In 2012, a team of Spanish engineers presented their idea for how a Titan boat would work, using propellers to put-put about across Titan’s seas. They called their mission the Titan Lake In-Situ Sampling Propelled Explorer, or TALISE.
Propellers are fine, but it turns out you could even have a sailboat on Titan. The methane seas have much less density and viscosity than water, which means that you’d only experience about 26% the friction of Earth. Cassini measured windspeeds of about 3.3 m/s across Titan, which half the average windspeed of Earth. But this would be plenty of wind to power a sail when you consider Titan’s thicker atmosphere.
And here’s my favorite idea. A submarine. This 6-meter vessel would float on Titan’s Kraken Mare sea, studying the chemistry of the oceans, measuring currents and tides, and mapping out the sea floor.
It would be capable of diving down beneath the waves for periods, studying interesting regions up close, and then returning to the surface to communicate its findings back to Earth. This mission is in the conceptual stage right now, but it was recently chosen by NASA’s Innovative Advanced Concepts Group for further study. If all goes well, the submarine would travel to Titan by 2038 when there’s a good planetary alignment.
Okay? Are you convinced? Let’s go back to Titan. Let’s explore it from the air, crawl around on the surface and dive beneath its waves. It’s one of the most interesting places in the entire Solar System, and we’ve only scratched the surface.
If I’ve done my job right, you’re as excited about a mission to Titan as I am. Let’s go back, let’s sail and submarine around that place. Let me know your thoughts in the comments.
How Do Volcanoes Erupt?
Volcanoes come in many shapes and sizes, ranging from common cinder cone volcanoes that build up from repeated eruptions and lava domes that pile up over volcanic vents to broad shield volcanoes and composite volcanoes. Though they differ in terms of structure and appearance, they all share two things. On the one hand, they are all awesome forces of nature that both terrify and inspire.
On the other, all volcanic activity comes down to the same basic principle. In essence, all eruptions are the result of magma from beneath the Earth being pushed up to the surface where it erupts as lava, ash and rock. But what mechanisms drive this process? What is it exactly that makes molten rock rise from the Earth’s interior and explode onto the landscape?
To understand how volcanoes erupt, one first needs to consider the structure of the Earth. At the very top is the lithosphere, the outermost layers of the Earth that consists of the upper mantle and crust. The crust makes up a tiny volume of the Earth, ranging from 10 km in thickness on the ocean floor to a maximum of 100 km in mountainous regions. It is cold and rigid, and composed primarily of silicate rock.
Beneath the crust, the Earth’s mantle is divided into sections of varying thickness based on their seismology. These consist of the upper mantle, which extends from a depth of 7 – 35 km (4.3 to 21.7 mi)) to 410 km (250 mi); the transition zone, which ranges from 410–660 km (250–410 mi); the lower mantle, which ranges from 660–2,891 km (410–1,796 mi); and the core–mantle boundary, which is ~200 km (120 mi) thick on average.
In the mantle region, conditions change drastically from the crust. Pressures increase considerably and temperatures can reach up to 1000 °C, which makes the rock viscous enough that it behaves like a liquid. In short, it experiences elastically on time scales of thousands of years or greater. This viscous, molten rock collects into vast chambers beneath the Earth’s crust.
Since this magma is less dense than the surrounding rock, it ” floats” up to the surface, seeking out cracks and weaknesses in the mantle. When it finally reaches the surface, it explodes from the summit of a volcano. When it’s beneath the surface, the molten rock is called magma. When it reaches the surface, it erupts as lava, ash and volcanic rocks.
With each eruption, rocks, lava and ash build up around the volcanic vent. The nature of the eruption depends on the viscosity of the magma. When the lava flows easily, it can travel far and create wide shield volcanoes. When the lava is very thick, it creates a more familiar cone volcano shape (aka. a cinder cone volcano). When the lava is extremely thick, it can build up in the volcano and explode (lava domes).
Another mechanism that drives volcanism is the motion the crust undergoes. To break it down, the lithosphere is divided into several plates, which are constantly in motion atop the mantle. Sometimes the plates collide, pull apart, or slide alongside each other; resulting in convergent boundaries, divergent boundaries, and transform boundaries. This activity is what drives geological activity, which includes earthquakes and volcanoes.
In the case of the former, subduction zones are often the result, where the heavier plate slips under the lighter plate – forming a deep trench. This subduction changes the dense mantle into buoyant magma, which rises through the crust to the Earth’s surface. Over millions of years, this rising magma creates a series of active volcanoes known as a volcanic arc.
In short, volcanoes are driven by pressure and heat in the mantle, as well as tectonic activity that leads to volcanic eruptions and geological renewal. The prevalence of volcanic eruptions in certain regions of the world – such as the Pacific Ring of Fire – also has a profound impact on the local climate and geography. For example, such regions are generally mountainous, have rich soil, and periodically experience the formation of new landmasses.
We have written many articles about volcanoes here at Universe Today. Here’s What are the Different Types of Volcanoes?, What are the Different Parts of a Volcano?, 10 Interesting Facts About Volcanoes?, What is the Pacific Ring of Fire?, Olympus Mons: The Largest Volcano in the Solar System.
Want more resources on the Earth? Here’s a link to NASA’s Human Spaceflight page, and here’s NASA’s Visible Earth.
We have also recorded an episode of Astronomy Cast about Earth, as part of our tour through the Solar System – Episode 51: Earth.
What is the Difference Between Lava and Magma?
Few forces in nature are are impressive or frightening as a volcanic eruption. In an instant, from within the rumbling depths of the Earth, hot lava, steam, and even chunks of hot rock are spewed into the air, covering vast distances with fire and ash. And thanks to the efforts of geologists and Earth scientists over the course of many centuries, we have to come to understand a great deal about them.
However, when it comes to the nomenclature of volcanoes, a point of confusion often arises. Again and again, one of the most common questions about volcanoes is, what is the difference between lava and magma? They are both molten rock, and are both associated with volcanism. So why the separate names? As it turns out, it all comes down to location.
Earth’s Composition:
As anyone with a basic knowledge of geology will tell you, the insides of the Earth are very hot. As a terrestrial planet, its interior is differentiated between a molten, metal core, and a mantle and crust composed primarily of silicate rock. Life as we know it, consisting of all vegetation and land animals, live on the cool crust, whereas sea life inhabits the oceans that cover a large extent of this same crust.
However, the deeper one goes into the planet, both pressures and temperatures increase considerably. All told, Earth’s mantle extends to a depth of about 2,890 km, and is composed of silicate rocks that are rich in iron and magnesium relative to the overlying crust. Although solid, the high temperatures within the mantle cause pockets of molten rock to form.
This silicate material is less dense than the surrounding rock, and is therefore sufficiently ductile that it can flow on very long timescales. Over time, it will also reach the surface as geological forces push it upwards. This happens as a result of tectonic activity.
Basically, the cool, rigid crust is broken into pieces called tectonic plates. These plates are rigid segments that move in relation to one another at one of three types of plate boundaries. These are known as convergent boundaries, at which two plates come together; divergent boundaries, at which two plates are pulled apart; and transform boundaries, in which two plates slide past one another laterally.
Interactions between these plates are what is what is volcanic activity (best exemplified by the “Pacific Ring of Fire“) as well as mountain-building. As the tectonic plates migrate across the planet, the ocean floor is subducted – the leading edge of one plate pushing under another. At the same time, mantle material will push up at divergent boundaries, forcing molten rock to the surface.
Magma:
As already noted, both lava and magma are what results from rock superheated to the point where it becomes viscous and molten. But again, the location is the key. When this molten rock is still located within the Earth, it is known as magma. The name is derived from Greek, which translate to “thick unguent” (a word used to describe a viscous substance used for ointments or lubrication).
It is composed of molten or semi-molten rock, volatiles, solids (and sometimes crystals) that are found beneath the surface of the Earth. This vicious rock usually collects in a magma chamber beneath a volcano, or solidify underground to form an intrusion. Where it forms beneath a volcano, it can then be injected into cracks in rocks or issue out of volcanoes in eruptions. The temperature of magma ranges between 600 °C and 1600 °C.
Magma is also known to exist on other terrestrial planets in the Solar System (i.e. Mercury, Venus and Mars) as well as certain moons (Earth’s Moon and Jupiter’s moon Io). In addition to stable lava tubes being observed on Mercury, the Moon and Mars, powerful volcanoes have been observed on Io that are capable of sending lava jets 500 km (300 miles) into space.
Lava:
When magma reaches the surface and erupts from a volcano, it officially becomes lava. There are actually different kinds of lava depending on its thickness or viscosity. Whereas the thinnest lava can flow downhill for many kilometers (thus creating a gentle slope), thicker lavas will pile up around a volcanic vent and hardly flow at all. The thickest lava doesn’t even flow, and just plugs up the throat of a volcano, which in some cases cause violent explosions.
The term lava is usually used instead of lava flow. This describes a moving outpouring of lava, which occurs when a non-explosive effusive eruption takes place. Once a flow has stopped moving, the lava solidifies to form igneous rock. Although lava can be up to 100,000 times more viscous than water, lava can flow over great distances before cooling and solidifying.
The word “lava” comes from Italian, and is probably derived from the Latin word labes which means “a fall” or “slide”. The first use in connection with a volcanic event was apparently in a short written account by Franscesco Serao, who observed the eruption of Mount Vesuvius between May 14th and June 4th, 1737. Serao described “a flow of fiery lava” as an analogy to the flow of water and mud down the flanks of the volcano following heavy rain.
Such is the difference between magma and lava. It seems that in geology, as in real estate, its all about location!
We have written many articles about volcanoes here at Universe Today. Here’s What is Lava?, What is the Temperature of Lava?, Igneous Rocks: How Are They Formed?, What Are The Different Parts Of A Volcano? and Planet Earth.
Want more resources on the Earth? Here’s a link to NASA’s Human Spaceflight page, and here’s NASA’s Visible Earth.
We have also recorded an episode of Astronomy Cast about Earth, as part of our tour through the Solar System – Episode 51: Earth.
What Are The Benefits Of Volcanoes?
Volcanoes are renowned for their destructive power. In fact, there are few forces of nature that rival their sheer, awesome might, or have left as big of impact on the human psyche. Who hasn’t heard of tales of Mt. Vesuvius erupting and burying Pompeii? There’s also the Minoan Eruption, the eruption that took place in the 2nd millennium BCE on the isle of Santorini and devastated the Minoan settlement there.
In Japan, Hawaii, South American and all across the Pacific, there are countless instances of eruptions taking a terrible toll. And who can forget modern-day eruptions like Mount St. Helens? But would it surprise you to know that despite their destructive power, volcanoes actually come with their share of benefits? From enriching the soil to creating new landmasses, volcanoes are actually a productive force as well.
Soil Enrichment:
Volcanic eruptions result in ash being dispersed over wide areas around the eruption site. And depending on the chemistry of the magma from which it erupted, this ash will be contain varying amounts of soil nutrients. While the most abundant elements in magma are silica and oxygen, eruptions also result in the release of water, carbon dioxide (CO²), sulfur dioxide (SO²), hydrogen sulfide (H²S), and hydrogen chloride (HCl), amongst others.
In addition, eruptions release bits of rock such as potolivine, pyroxene, amphibole, and feldspar, which are in turn rich in iron, magnesium, and potassium. As a result, regions that have large deposits of volcanic soil (i.e. mountain slopes and valleys near eruption sites) are quite fertile. For example, most of Italy has poor soils that consist of limestone rock.
But in the regions around Naples (the site of Mt. Vesuvius), there are fertile stretches of land that were created by volcanic eruptions that took place 35,000 and 12,000 years ago. The soil in this region is rich because volcanic eruption deposit the necessary minerals, which are then weathered and broken down by rain. Once absorbed into the soil, they become a steady supply of nutrients for plant life.
Hawaii is another location where volcanism led to rich soil, which in turn allowed for the emergence of thriving agricultural communities. Between the 15th and 18th centuries on the islands of Kauai, O’ahu and Molokai, the cultivation of crops like taros and sweet potatoes allowed for the rise of powerful chiefdoms and the flowering of the culture we associate with Hawaii today.
Volcanic Land Formations:
In addition to scattering ash over large areas of land, volcanoes also push material to the surface that can result in the formation of new islands. For example, the entire Hawaiian chain of islands was created by the constant eruptions of a single volcanic hot spot. Over hundreds of thousands of years, these volcanoes breached the surface of the ocean becoming habitable islands, and rest stops during long sea journeys.
This is the case all across the Pacific, were island chains such as Micronesia, the Ryukyu Islands (between Taiwan and Japan), the Aleutian Islands (off the coast of Alaska), the Mariana Islands, and Bismark Archipelago were all formed along arcs that are parallel and close to a boundary between two converging tectonic plates.
Much the same is true of the Mediterranean. Along the Hellenic Arc (in the eastern Mediterranean), volcanic eruptions led to the creation of the Ionian Islands, Cyprus and Crete. The nearby South Aegean Arc meanwhile led to the formation of Aegina, Methana, Milos, Santorini and Kolumbo, and Kos, Nisyros and Yali. And in the Caribbean, volcanic activity led to the creation of the Antilles archipelago.
Where these islands formed, unique species of plants and animals evolved into new forms on these islands, creating balanced ecosystems and leading to new levels of biodiversity.
Volcanic Minerals and Stones:
Another benefits to volcanoes are the precious gems, minerals and building materials that eruptions make available. For instance, stones like pumice volcanic ash and perlite (volcanic glass) are all mined for various commercial uses. These include acting as abrasives in soaps and household cleaners. Volcanic ash and pumice are also used as a light-weight aggregate for making cement.
The finest grades of these volcanic rocks are used in metal polishes and for woodworking. Crushed and ground pumice are also used for loose-fill insulation, filter aids, poultry litter, soil conditioner, sweeping compound, insecticide carrier, and blacktop highway dressing.
Perlite is also used as an aggregate in plaster, since it expands rapidly when heated. In precast walls, it too is used as an aggregate in concrete. Crushed basalt and diasbase are also used for road metal, railroad ballast, roofing granules, or as protective arrangements for shorelines (riprap). High-density basalt and diabase aggregate are used in the concrete shields of nuclear reactors.
Hardened volcanic ash (called tuff) makes an especially strong, lightweight building material. The ancient Romans combined tuff and lime to make a strong, lightweight concrete for walls, and buildings. The roof of the Pantheon in Rome is made of this very type of concrete because it’s so lightweight.
Precious metals that are often found in volcanoes include sulfur, zinc, silver, copper, gold, and uranium. These metals have a wide range of uses in modern economies, ranging from fine metalwork, machinery and electronics to nuclear power, research and medicine. Precious stones and minerals that are found in volcanoes include opals, obsidian, fire agate, flourite, gypsum, onyx, hematite, and others.
Global Cooling:
Volcanoes also play a vital role in periodically cooling off the planet. When volcanic ash and compounds like sulfur dioxide are released into the atmosphere, it can reflect some of the Sun’s rays back into space, thereby reducing the amount of heat energy absorbed by the atmosphere. This process, known as “global dimming”, therefore has a cooling effect on the planet.
The link between volcanic eruptions and global cooling has been the subject of scientific study for decades. In that time, several dips have been observed in global temperatures after large eruptions. And though most ash clouds dissipate quickly, the occasional prolonged period of cooler temperatures have been traced to particularly large eruptions.
Because of this well-established link, some scientists have recommended that sulfur dioxide and other be released into the atmosphere in order to combat global warming, a process which is known as ecological engineering.
Hot Springs And Geothermal Energy:
Another benefit of volcanism comes in the form of geothermal fields, which is an area of the Earth characterized by a relatively high heat flow. These fields, which are the result of present, or fairly recent magmatic activity, come in two forms. Low temperature fields (20-100°C) are due to hot rock below active faults, while high temperature fields (above 100°C) are associated with active volcanism.
Geothermal fields often create hot springs, geysers and boiling mud pools, which are often a popular destination for tourists. But they can also be harnessed for geothermal energy, a form of carbon-neutral power where pipes are placed in the Earth and channel steam upwards to turn turbines and generate electricity.
In countries like Kenya, Iceland, New Zealand, the Phillipines, Costa Rica and El Salvador, geothermal power is responsible for providing a significant portion of the country’s power supply – ranging from 14% in Costa Rica to 51% in Kenya. In all cases, this is due to the countries being in and around active volcanic regions that allow for the presence of abundant geothermal fields.
Outgassing and Atmospheric Formation:
But by far, the most beneficial aspect of volcanoes is the role they play in the formation of a planet’s atmosphere. In short, Earth’s atmosphere began to form after its formation 4.6 billion eyars ago, when volcanic outgassing led to the creation of gases stored in the Earth’s interior to collect around the surface of the planet. Initially, this atmosphere consisted of hydrogen sulfide, methane, and 10 to 200 times as much carbon dioxide as today’s atmosphere.
After about half a billion years, Earth’s surface cooled and solidified enough for water to collect on it. At this point, the atmosphere shifted to one composed of water vapor, carbon dioxide and ammonia (NH³). Much of the carbon dioxide dissolved into the oceans, where cyanobacteria developed to consume it and release oxygen as a byproduct. Meanwhile, the ammonia began to be broken down by photolysis, releasing the hydrogen into space and leaving the nitrogen behind.
Another key role played by volcanism occurred 2.5 billion years ago, during the boundary between the Archaean and Proterozoic Eras. It was at this point that oxygen began to appear in our oxygen due to photosynthesis – which is referred to asthe “Great Oxidation Event”. However, according to recent geological studies, biomarkers indicate that oxygen-producing cyanobacteria were releasing oxygen at the same levels there are today. In short, the oxygen being produced had to be going somewhere for it not to appear in the atmosphere.
The lack of terrestrial volcanoes is believed to be responsible. During the Archaean Era, there were only submarine volcanoes, which had the effect of scrubbing oxygen from the atmosphere, binding it into oxygen containing minerals. By the Archaean/Proterozoic boundary, stabilized continental land masses arose, leading to terrestrial volcanoes. From this point onward, markers show that oxygen began appearing in the atmosphere.
Volcanism also plays a vital role in the atmospheres of other planets. Mercury’s thin exosphere of hydrogen, helium, oxygen, sodium, calcium, potassium and water vapor is due in part of volcanism, which periodically replenishes it. Venus’ incredibly dense atmosphere is also believed to be periodically replenished by volcanoes on its surface.
And Io, Jupiter’s volcanically active moon, has an extremely tenuous atmosphere of sulfur dioxide (SO²), sulfur monoxide (SO), sodium chloride (NaCl), sulfur monoxide (SO), atomic sulfur (S) and oxygen (O). All of these gases are provided and replenished by the many hundreds of volcanoes situated across the moon’s surface.
As you can see, volcanoes are actually a pretty creative force when all is said and done. In fact, us terrestrial organisms depend on them for everything from the air we breathe, to the rich soil that produces our food, to the geological activity that gives rise to terrestrial renewal and biological diversity.
We have written many articles about volcanoes for Universe Today. Here’s an article about extinct volcanoes, and here’s an article about active volcanoes. Here’s an article about volcanoes.
Want more resources on the Earth? Here’s a link to NASA’s Human Spaceflight page, and here’s NASA’s Visible Earth.
Astronomy Cast also has relevant episodes on the subject Earth, as part of our tour through the Solar System – Episode 51: Earth.
Mountains: How Are They Formed?
When beholding the sheer size and majesty of mountains, ancient humans could not help but feel that they were standing in the presence of something… godlike. And within the belief systems of many ancient cultures, it was generally felt that mountains were something spiritual – either serving as the home of the Gods, a result of their activity, or a place to get closer to God.
Thanks to modern geology, we now know the true story of how mountains are formed. Simply put, they are the result of tectonic forces or volcanism. But knowing this has not diminished their impressive and awe-inspiring nature. When a geological formation is created through forces that can only be described as titanic, this is to be expected. But just how are mountains formed?
In truth, there are three ways in which mountains are formed, which correspond to the types of mountains in question. These are known as volcanic, fold and block mountains. All of these are the result of plate tectonics, where compressional forces, isostatic uplift and intrusion of igneous matter forces surface rock upward, creating a landform higher than the surrounding features.
Over the course of many million years, these uplifted sections are eroded by the elements – wind, rain, ice and gravity. These gradually wear the surface of the mountains down, cause the surface to be younger than the rocks that form them, and lead to the types of formations and distributions we are familiar with today.
Volcanic Mountains:
Volcanic mountains are formed when a tectonic plate is pushed beneath another (or above a mid-ocean ridge or hotspot) where magma is forced to the surface. When the magma reaches the surface, it often builds a volcanic mountain, such as s shield volcano or a stratovolcano. Examples of this sort of mountains include Mount Fuji in Japan, Mauna Kea in Hawaii, Nyamuragira in the Democratic Republic of Congo, Skjaldbreiður in Iceland and Mount Etna in Sicily.
At other times, the rising magma solidifies below the surface and forms dome mountains, where material is pushed up from the force of the build-up beneath it. Examples of this formation include Navajo Mountain in San Juan County, Utah; the Chaitén lava dome of Chile, Torfajökull in Iceland, and Mount St. Helens in Washington State.
Fold Mountains:
As the name suggests, fold mountains occur when two tectonic plates collide at a convergent plate boundary, causing the crust to overthicken. This process forces the less dense crust to float on top of the denser mantle rocks – with material being forced upwards to form hills, plateaus or mountains – while a greater volume of material is forced downward into the mantle.
The Jura Mountains, a series of sub-parallel mountain ridges located in the Alps, are an example of fold mountains. Other examples include the “Simply Folded Belt” of the Zagros mountains, which extends from northern Syria and southern Turkey to eastern Iran and the Persian Gulf. There is also the Akwapim-Togo ranges in Ghana and the Ridge-and-Valley Appalachians in the Eastern United States.
But perhaps most famous is the Himalayan mountain chain, located between northern India and Nepal. This chain formed as a result of the collision between the Indian subcontinent and Asia some 25 million years ago, and has given rise to the tallest mountain in the world – Mt. Everest.
Block Mountains:
Block mountains are caused by faults in the crust, a seam where rocks can move past each other. Also known as rifting, this process occurs when rocks on one side of a fault rise relative to the other. The uplifted blocks become block mountains (also known as horsts) while the intervening dropped blocks are known as graben (i.e. depressed regions).
Examples of this type of terrain can be found in the Upper Rhine valley, the Vosges mountains in France, the Black Forest in Germany, and the Vindhya and Satpura horsts in India. There is also the East African Rift, an active continental rift zone with several active volcanoes that extends from Eritrea to Mozambique.
Mountain Erosion:
As noted, the final way in which mountains are formed is through erosion. This occurs during and after an uplift, where a newly formed mountainous region is subjected to the effects of wind, water, ice, and gravity. These forces actively shape the surface of mountain ranges, wearing down the exposed surfaces, depositing sediment in alluvial flows, and leading to the formation of characteristic landforms.
These include pyramidal peaks, knife-edge arêtes, and bowl-shaped cirques that can contain lakes. Plateau mountains, such as the Catskills, are formed from the erosion of an uplifted plateau. And after millions of years of erosion, mountains may cease to exist entirely.
Given the size and scale of a mountain, the immense forces involved in their creation, and the immense amount of time it takes to shape and form them, it is little wonder why they are considered such a big deal. Between their religious significance (i.e. Mount Zion, Mount Olympus, Mount Ararat, and Mauna Kea, to name a few), their scenic value, the challenge they present, and their importance to the Earth sciences, these geological formations continue to enjoy a special place in our hearts, minds and culture.
As we explore other planets, we have also found new and impressive mountain formations that have taught us much about the geological activity and composition of other worlds. For example, there the volcanic mountain on Mars known as Olympus Mons, which just happens to be the largest mountain in the Solar System. And this is merely a drop in the bucket. Wherever there’s a geologically active planet, there’s mountains to be found!
We have written many articles about mountains here at Universe Today. Here’s one on Fault-Block Mountains, one on Volcanic Mountains, one on Fold Mountains, and one on Dome Mountains. And here’s an article about The Clearest Skies On Earth.
For more information, check out NASA’s Human Spaceflight page, and here’s NASA’s Visible Earth.
Astronomy Cast also has a great episode on the subject – Episode 51: Earth.
What Are The Different Parts Of A Volcano?
Without a doubt, volcanoes are one of the most powerful forces of nature a person can bear witness to. Put simply, they are what results when a massive rupture takes place in the Earth’s crust (or any planetary-mass object), spewing hot lava, volcanic ash, and toxic fumes onto the surface and air. Originating from deep within the Earth’s crust, volcanoes leave a lasting mark on the landscape.
But what are the specific parts of a volcano? Aside from the “volcanic cone” (i.e. the cone-shaped mountain), a volcano has many different parts and layers, most of which are located within the mountainous region or deep within the Earth. As such, any true understanding of their makeup requires that we do a little digging (so to speak!)
While volcanoes come in a number of shapes and sizes, certain common elements can be discerned. The following gives you a general breakdown of a volcanoes specific parts, and what goes into making them such a titanic and awesome natural force.
Magma Chamber:
A magma chamber is a large underground pool of molten rock sitting underneath the Earth’s crust. The molten rock in such a chamber is under extreme pressure, which in time can lead to the surrounding rock fracturing, creating outlets for the magma. This, combined with the fact that the magma is less dense than the surrounding mantle, allows it to seep up to the surface through the mantle’s cracks.
When it reaches the surface, it results in a volcanic eruption. Hence why many volcanoes are located above a magma chamber. Most known magma chambers are located close to the Earth’s surface, usually between 1 km and 10 km deep. In geological terms, this makes them part of the Earth’s crust – which ranges from 5–70 km (~3–44 miles) deep.
Lava:
Lava is the silicate rock that is hot enough to be in liquid form, and which is expelled from a volcano during an eruption. The source of the heat that melts the rock is known as geothermal energy – i.e. heat generated within the Earth that is leftover from its formation and the decay of radioactive elements. When lava first erupted from a volcanic vent (see below), it comes out with a temperature of anywhere between 700 to 1,200 °C (1,292 to 2,192 °F). As it makes contact with air and flows downhill, it eventually cools and hardens.
Main Vent:
A volcano’s main vent is the weak point in the Earth’s crust where hot magma has been able to rise from the magma chamber and reach the surface. The familiar cone-shape of many volcanoes are an indication of this, the point at which ash, rock and lava ejected during an eruption fall back to Earth around the vent to form a protrusion.
Throat:
The uppermost section of the main vent is known as the volcano’s throat. As the entrance to the volcano, it is from here that lava and volcanic ash are ejected.
Crater:
In addition to cone structures, volcanic activity can also lead to circular depressions (aka. craters) forming in the Earth. A volcanic crater is typically a basin, circular in form, which can be large in radius and sometimes great in depth. In these cases, the lava vent is located at the bottom of the crater. They are formed during certain types of climactic eruptions, where the volcano’s magma chamber empties enough for the area above it to collapse, forming what is known as a caldera.
Pyroclastic Flow:
Otherwise known as a pyroclastic density current, a pyroclastic flow refers to a fast-moving current of hot gas and rock that is moving away from a volcano. Such flows can reach speeds of up to 700 km/h (450 mph), with the gas reaching temperatures of about 1,000 °C (1,830 °F). Pyroclastic flows normally hug the ground and travel downhill from their eruption site.
Their speeds depend upon the density of the current, the volcanic output rate, and the gradient of the slope. Given their speed, temperature, and the way they flow downhill, they are one of the greatest dangers associated with volcanic eruptions and are one of the primary causes of damage to structures and the local environment around an eruption site.
Ash Cloud:
Volcanic ash consists of small pieces of pulverized rock, minerals and volcanic glass created during a volcanic eruption. These fragments are generally very small, measuring less than 2 mm (0.079 inches) in diameter. This sort of ash forms as a result of volcanic explosions, where dissolved gases in magma expand to the point where the magma shatters and is propelled into the atmosphere. The bits of magma then cool, solidifying into fragments of volcanic rock and glass.
Because of their size and the explosive force with which they are generated, volcanic ash is picked up by winds and dispersed up to several kilometers away from the eruption site. Due to this dispersal, ash an also have a damaging effect on the local environment, which includes negatively affecting human and animal health, disrupting aviation, disrupting infrastructure, and damaging agriculture and water systems. Ash is also produced when magma comes into contact with water, which causes the water to explosively evaporate into steam and for the magma to shatter.
Volcanic Bombs:
In addition to ash, volcanic eruptions have also been known to send larger projectiles flying through the air. Known as volcanic bombs, these ejecta are defined as those that measure more than 64mm (2.5 inches) in diameter, and which are formed when a volcano ejects viscous fragments of lava during an eruption. These cool before they hit the ground, are thrown many kilometers from the eruption site, and often acquire aerodynamic shapes (i.e. streamlined in form).
While the term applies to any ejecta larger than a few centimeters, volcanic bombs can sometimes be very large. There have been recorded instances where objects measuring several meters were retrieved hundreds of meters from an eruptions. Small or large, volcanic bombs are a significant volcanic hazard and can often cause serious damage and multiple fatalities, depending on where they land. Luckily, such explosions are rare.
Secondary Vent:
On large volcanoes, magma can reach the surface through several different vents. Where they reach the surface of the volcano, they form what is referred to as a secondary vent. Where they are interrupted by accumulated ash and solidified lava, they become what is known as a Dike. And where these intrude between cracks, pool and then crystallize, they form what is called a Sill.
Secondary Cone:
Also known as a Parasitic Cone, secondary cones build up around secondary vents that reach the surface on larger volcanoes. As they deposit lava and ash on the exterior, they form a smaller cone, one that resembles a horn on the main cone.
Yes indeed, volcanoes are as powerful as they are dangerous. And yet, without these geological phenomena occasionally breaking through the surface and reigning down fire, smoke, and clouds of ash, the world as we know it would be a very different place. More than likely, it would be a geologically dead one, with no change or evolution in its crust. I think we can all agree that while such a world would be much safer, it would also be painfully boring!
We have written many interesting articles about volcanoes here at Universe Today. Here’s is one about the different types of volcanoes, one about composite volcanoes, and here’s one on the famous volcanic belt, the Pacific “Ring of Fire”.
Astronomy Cast also has a lovely episodes about volcanoes and geology, titled Episode 307: Pacific Ring of Fire and Episode 51: Earth
Want more resources on the Earth? Here’s a link to NASA’s Human Spaceflight page, and here’s NASA’s Visible Earth.